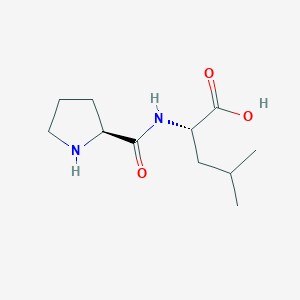
Pro-leu
Overview
Description
Pro-Leu is a synthetic peptide derived from the amino acid leucine. It is a powerful and versatile molecule that has been used in a variety of scientific and medical applications. In recent years, Pro-Leu has become increasingly popular in laboratory experiments due to its ability to induce cell growth, facilitate gene expression, and promote cell differentiation.
Scientific Research Applications
Food Science: Bitterness Suppression
“H-Pro-Leu-OH” has been studied for its sensory properties, particularly in the context of bitterness suppression. A heptapeptide, which includes the sequence of H-Pro-Leu-OH, has been synthesized to measure its threshold value of bitterness, which is a crucial aspect in food science for flavor enhancement and bitterness masking .
Antioxidant Applications
Peptides containing the H-Pro-Leu-OH sequence have been identified as potential antioxidants. These peptides can be used to restore oxidative stress-induced damage in cellular models, indicating their excellent antioxidant capacity. This application is significant in the development of therapeutic agents and nutritional supplements .
Material Science: Additive Engineering
The compound’s structural characteristics allow it to be used in material science, particularly in additive engineering. It can modulate crystallization processes and improve charge carrier transport in semiconductor applications .
Mechanism of Action
Target of Action
H-Pro-Leu-OH, also known as Pro-leu or (S)-4-Methyl-2-((S)-pyrrolidine-2-carboxamido)pentanoic acid, primarily targets the human kidney prolinase . Prolinase, also known as prolyl dipeptidase, is an enzyme that plays a crucial role in the metabolism of proline-containing peptides .
Mode of Action
It is known that h-pro-leu-oh acts as a substrate for this enzyme . This suggests that the compound may be involved in the enzymatic processes mediated by prolinase, potentially influencing the metabolism of proline-containing peptides.
properties
IUPAC Name |
(2S)-4-methyl-2-[[(2S)-pyrrolidine-2-carbonyl]amino]pentanoic acid | |
---|---|---|
Source | PubChem | |
URL | https://pubchem.ncbi.nlm.nih.gov | |
Description | Data deposited in or computed by PubChem | |
InChI |
InChI=1S/C11H20N2O3/c1-7(2)6-9(11(15)16)13-10(14)8-4-3-5-12-8/h7-9,12H,3-6H2,1-2H3,(H,13,14)(H,15,16)/t8-,9-/m0/s1 | |
Source | PubChem | |
URL | https://pubchem.ncbi.nlm.nih.gov | |
Description | Data deposited in or computed by PubChem | |
InChI Key |
ZKQOUHVVXABNDG-IUCAKERBSA-N | |
Source | PubChem | |
URL | https://pubchem.ncbi.nlm.nih.gov | |
Description | Data deposited in or computed by PubChem | |
Canonical SMILES |
CC(C)CC(C(=O)O)NC(=O)C1CCCN1 | |
Source | PubChem | |
URL | https://pubchem.ncbi.nlm.nih.gov | |
Description | Data deposited in or computed by PubChem | |
Isomeric SMILES |
CC(C)C[C@@H](C(=O)O)NC(=O)[C@@H]1CCCN1 | |
Source | PubChem | |
URL | https://pubchem.ncbi.nlm.nih.gov | |
Description | Data deposited in or computed by PubChem | |
Molecular Formula |
C11H20N2O3 | |
Source | PubChem | |
URL | https://pubchem.ncbi.nlm.nih.gov | |
Description | Data deposited in or computed by PubChem | |
Molecular Weight |
228.29 g/mol | |
Source | PubChem | |
URL | https://pubchem.ncbi.nlm.nih.gov | |
Description | Data deposited in or computed by PubChem | |
Product Name |
Pro-leu | |
CAS RN |
52899-07-7 | |
Record name | L-Prolyl-L-leucine | |
Source | CAS Common Chemistry | |
URL | https://commonchemistry.cas.org/detail?cas_rn=52899-07-7 | |
Description | CAS Common Chemistry is an open community resource for accessing chemical information. Nearly 500,000 chemical substances from CAS REGISTRY cover areas of community interest, including common and frequently regulated chemicals, and those relevant to high school and undergraduate chemistry classes. This chemical information, curated by our expert scientists, is provided in alignment with our mission as a division of the American Chemical Society. | |
Explanation | The data from CAS Common Chemistry is provided under a CC-BY-NC 4.0 license, unless otherwise stated. | |
Retrosynthesis Analysis
AI-Powered Synthesis Planning: Our tool employs the Template_relevance Pistachio, Template_relevance Bkms_metabolic, Template_relevance Pistachio_ringbreaker, Template_relevance Reaxys, Template_relevance Reaxys_biocatalysis model, leveraging a vast database of chemical reactions to predict feasible synthetic routes.
One-Step Synthesis Focus: Specifically designed for one-step synthesis, it provides concise and direct routes for your target compounds, streamlining the synthesis process.
Accurate Predictions: Utilizing the extensive PISTACHIO, BKMS_METABOLIC, PISTACHIO_RINGBREAKER, REAXYS, REAXYS_BIOCATALYSIS database, our tool offers high-accuracy predictions, reflecting the latest in chemical research and data.
Strategy Settings
Precursor scoring | Relevance Heuristic |
---|---|
Min. plausibility | 0.01 |
Model | Template_relevance |
Template Set | Pistachio/Bkms_metabolic/Pistachio_ringbreaker/Reaxys/Reaxys_biocatalysis |
Top-N result to add to graph | 6 |
Feasible Synthetic Routes
Q & A
Q1: What is the molecular formula and weight of Pro-Leu (H-Pro-Leu-OH)?
A1: The molecular formula of Pro-Leu (H-Pro-Leu-OH) is C11H20N2O3, and its molecular weight is 228.29 g/mol.
Q2: Is there any spectroscopic data available for Pro-Leu (H-Pro-Leu-OH)?
A2: Yes, several research papers utilize techniques like Nuclear Magnetic Resonance (NMR) spectroscopy and Circular Dichroism (CD) spectroscopy to analyze Pro-Leu-containing peptides. For instance, one study employed 1H NMR and CD to investigate the conformation of cyclo(Pro-Leu-Aib)2, a cyclic peptide containing the Pro-Leu motif [].
Q3: How does modifying the Pro-Leu (H-Pro-Leu-OH) structure affect its biological activity?
A3: Research suggests that even minor alterations to the Pro-Leu structure can significantly impact its biological activity. For example, replacing the proline residue with other heterocyclic amino acids can influence the peptide's ability to modulate dopamine receptors []. Similarly, modifying the C-terminal with cyclic amino acids can lead to varying levels of enhancement in dopamine agonist binding [].
Q4: Are there specific structural features of Pro-Leu analogs that correlate with enhanced activity in inhibiting oxotremorine-induced tremor?
A4: Yes, research indicates that while replacing Pro with HCO-Pro or cyclopentanecarboxylic acid leads to inactive analogs, peptides with the general structure <Glu-Leu-Gly-NR1R2 exhibit significant activity. For instance, R1 = C3H8 and R2 = H resulted in an analog four times more potent than MIF [].
Q5: Is Pro-Leu (H-Pro-Leu-OH) susceptible to enzymatic degradation?
A5: Yes, research indicates that Pro-Leu can be cleaved by enzymes. For example, one study demonstrated that Pro-Leu is a product of the enzymatic breakdown of the tripeptide Pro-Gly-Pro-Leu by enzymes found in rat nasal slime, brain microsomal fractions, and blood [].
Q6: How does the stability of simple glyprolines, including Pro-Leu, compare to that of Semax (Met-Glu-His-Phe-Pro-Gly-Pro)?
A6: Studies reveal that simple glyprolines like Pro-Gly-Pro-Leu, Pro-Gly-Pro-Gly, and Pro-Gly-Pro demonstrate significantly higher resistance to degradation by enzymes like leucine aminopeptidase and those found in nasal slime, brain membranes, and rat blood compared to Semax [].
Q7: What are some potential applications of Pro-Leu-containing peptides?
A7: Research suggests that Pro-Leu-containing peptides, specifically analogs of Pro-Leu-Gly-NH2 (MIF), may have potential in treating conditions like Parkinson's disease and mental depression due to their ability to inhibit oxotremorine-induced tremors [].
Q8: Does Pro-Leu (H-Pro-Leu-OH) play a role in the glycosylation of human gonadotropin alpha-subunit?
A8: Studies indicate that the Pro-Leu-Arg motif, while known to be involved in the glycosylation process, does not directly influence the sulfation of N-linked oligosaccharides in the human gonadotropin alpha-subunit [].
Q9: Can Pro-Leu-containing peptides inhibit collagenase activity?
A9: Yes, research demonstrates that synthetic peptides with the general structure Pro-Leu-X-Pro, where X can be glycine or sarcosine, can inhibit the collagenase enzyme from Achromobacter iophagus [].
Q10: Have computational methods been used to study Pro-Leu-containing peptides?
A10: Yes, conformational energy calculations have been employed to determine the tertiary structure of H-Pro-Leu-Gly-NH2, a peptide with MSH release-inhibiting properties []. Additionally, molecular modeling and simulations can be utilized to study the structure-activity relationship of Pro-Leu analogs and design more potent and selective compounds.
Q11: What is known about the distribution and half-life of Pro-Leu-Gly-NH2 in rats?
A11: Research shows that H-Pro-Leu-Gly-NH2, when labeled with 14C-leucine and administered intravenously to rats, exhibits a half-life of approximately 9 minutes and a distribution volume exceeding that of plasma [].
Q12: Does the source of protein in sheep feed impact the concentration of small peptides, including Pro-Leu, in the rumen?
A12: Yes, studies demonstrate a correlation between protein source and small peptide concentration in the sheep rumen. For example, a casein-rich diet led to the highest accumulation of Pro-Leu in the rumen 6 hours post-feeding [].
Disclaimer and Information on In-Vitro Research Products
Please be aware that all articles and product information presented on BenchChem are intended solely for informational purposes. The products available for purchase on BenchChem are specifically designed for in-vitro studies, which are conducted outside of living organisms. In-vitro studies, derived from the Latin term "in glass," involve experiments performed in controlled laboratory settings using cells or tissues. It is important to note that these products are not categorized as medicines or drugs, and they have not received approval from the FDA for the prevention, treatment, or cure of any medical condition, ailment, or disease. We must emphasize that any form of bodily introduction of these products into humans or animals is strictly prohibited by law. It is essential to adhere to these guidelines to ensure compliance with legal and ethical standards in research and experimentation.