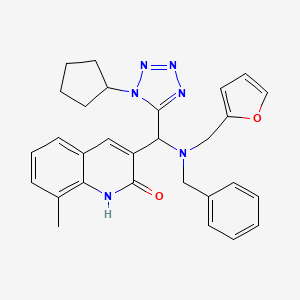
N,N-Diethyl-9H-purin-2-amine
Overview
Description
Preparation Methods
Synthetic Routes and Reaction Conditions
The synthesis of N,N-Diethyl-9H-purin-2-amine can be achieved through various methods. One common approach involves the alkylation of 9H-purin-2-amine with diethyl sulfate under basic conditions. The reaction typically proceeds as follows:
Starting Material: 9H-purin-2-amine
Reagent: Diethyl sulfate
Conditions: Basic medium (e.g., sodium hydroxide)
Product: this compound
Industrial Production Methods
In industrial settings, the production of this compound often involves the extraction and purification of caffeine from natural sources such as coffee beans and tea leaves. The process includes several steps:
Extraction: Using solvents like water or organic solvents to extract caffeine from plant material.
Purification: Employing techniques such as crystallization, filtration, and drying to obtain pure caffeine.
Chemical Reactions Analysis
Types of Reactions
N,N-Diethyl-9H-purin-2-amine undergoes various chemical reactions, including:
Oxidation: Caffeine can be oxidized to form various products, including theobromine and theophylline.
Reduction: Reduction of caffeine can yield compounds such as paraxanthine.
Substitution: Caffeine can undergo substitution reactions, particularly at the nitrogen atoms.
Common Reagents and Conditions
Oxidation: Reagents like potassium permanganate or hydrogen peroxide under acidic or basic conditions.
Reduction: Reagents such as lithium aluminum hydride or catalytic hydrogenation.
Substitution: Reagents like alkyl halides or acyl chlorides under basic conditions.
Major Products
Oxidation: Theobromine, theophylline
Reduction: Paraxanthine
Substitution: Various N-alkyl or N-acyl derivatives
Scientific Research Applications
N,N-Diethyl-9H-purin-2-amine has numerous applications in scientific research:
Chemistry: Used as a model compound in studies of alkaloid chemistry and reaction mechanisms.
Biology: Investigated for its effects on cellular metabolism and enzyme activity.
Medicine: Studied for its potential therapeutic effects, including its role as a stimulant and its impact on cognitive function.
Industry: Utilized in the food and beverage industry as a flavoring agent and stimulant.
Mechanism of Action
N,N-Diethyl-9H-purin-2-amine exerts its effects primarily by antagonizing adenosine receptors in the central nervous system. By blocking these receptors, caffeine prevents the inhibitory effects of adenosine, leading to increased neuronal activity and the release of neurotransmitters such as dopamine and norepinephrine . This results in heightened alertness, reduced fatigue, and improved cognitive function.
Comparison with Similar Compounds
Similar Compounds
Theobromine: Found in cocoa and chocolate, has similar stimulant effects but is less potent than caffeine.
Theophylline: Found in tea, used medically as a bronchodilator.
Paraxanthine: A metabolite of caffeine with similar stimulant properties.
Uniqueness
N,N-Diethyl-9H-purin-2-amine is unique due to its widespread consumption and well-documented effects on the central nervous system. Its ability to cross the blood-brain barrier and its relatively short half-life make it an effective and popular stimulant.
Biological Activity
N,N-Diethyl-9H-purin-2-amine, a purine derivative, has garnered attention in the field of medicinal chemistry due to its significant biological activities. This article delves into its biological mechanisms, therapeutic potential, and relevant case studies, supported by data tables and research findings.
Chemical Structure and Properties
This compound is characterized by a purine backbone with diethyl substitutions at the nitrogen atom. Its molecular formula is C₁₃H₁₈N₄, with a molecular weight of approximately 246.31 g/mol. The compound's structure facilitates its interaction with various biological targets, particularly enzymes involved in cell signaling and proliferation.
1. Enzyme Inhibition
Research has demonstrated that this compound exhibits inhibitory activity against several kinases, notably Nek2 (NIMA-related kinase 2). This kinase plays a critical role in cell cycle regulation, particularly during mitosis. The compound's selectivity for Nek2 over other kinases, such as CDK2 (cyclin-dependent kinase 2), suggests its potential as a targeted therapeutic agent for cancer treatment .
2. Interaction with Topoisomerases
The compound also interacts with topoisomerases, enzymes essential for DNA replication and transcription. Inhibition of these enzymes can disrupt DNA processes, leading to apoptosis in rapidly dividing cells. This mechanism is particularly relevant in the context of cancer therapy, where targeting proliferative pathways is crucial.
Table 1: Summary of Biological Activities
Activity | Target | IC50 Value (μM) | Comments |
---|---|---|---|
Nek2 Inhibition | Nek2 | 0.62 | High selectivity over CDK2 |
Topoisomerase Inhibition | Topoisomerase I & II | Varies | Disrupts DNA replication |
Cytotoxicity | Various Cancer Cell Lines | IC50 11.32 - 29.10 | Effective against glioma and colon carcinoma |
Case Study: Selective Inhibition of Nek2
In a study examining the selectivity of this compound for Nek2, it was found that the compound had an IC50 value of 0.62 μM for Nek2 while exhibiting significantly higher values for CDK2 (7.0 μM) . This selectivity is crucial for minimizing off-target effects associated with broader-spectrum kinase inhibitors.
Case Study: Cytotoxic Effects on Cancer Cells
Another study highlighted the cytotoxic effects of this compound on various cancer cell lines, including U87MG (human glioblastoma) and HCT116 (human colon carcinoma). The compound demonstrated IC50 values ranging from 11.32 to 29.10 μM, indicating its potential as an anti-cancer agent .
Potential Applications
The unique properties of this compound position it as a promising candidate for drug development in oncology. Its ability to selectively inhibit key kinases involved in cell cycle regulation opens avenues for targeted therapies that could reduce the side effects commonly associated with conventional chemotherapy.
Q & A
Basic Research Questions
Q. What are the key considerations for optimizing the synthesis of N,N-Diethyl-9H-purin-2-amine to maximize yield and purity?
Methodological Answer: Synthesis optimization requires attention to:
- Reaction Conditions : Temperature control (e.g., 60–80°C for alkylation) and solvent selection (polar aprotic solvents like DMF enhance nucleophilic substitution).
- Purification : Column chromatography with gradients of ethyl acetate/hexane or methanol/dichloromethane to isolate the product from by-products (e.g., unreacted amines or chlorinated intermediates) .
- Catalysts : Use of mild bases (e.g., K₂CO₃) to deprotonate intermediates without inducing side reactions .
- Monitoring : TLC or HPLC to track reaction progress and ensure completion before quenching.
Q. Which spectroscopic and crystallographic techniques are most effective for characterizing this compound?
Methodological Answer:
- Nuclear Magnetic Resonance (NMR) :
- X-ray Crystallography : Use SHELX programs (e.g., SHELXL) for structure refinement. Ensure high-resolution data collection (<1.0 Å) to resolve tautomeric forms and hydrogen bonding .
- Mass Spectrometry (HRMS) : Confirm molecular formula via exact mass matching (e.g., [M+H]⁺ ion).
Advanced Research Questions
Q. How can computational modeling predict the reactivity and tautomeric behavior of this compound?
Methodological Answer:
- Density Functional Theory (DFT) : Calculate energy minima for tautomers (e.g., N9-H vs. N7-H forms) using Gaussian or ORCA. Compare bond lengths and angles to crystallographic data .
- Molecular Dynamics (MD) : Simulate solvation effects (e.g., in DMSO or water) to predict dominant tautomers under physiological conditions.
- Docking Studies : Map interactions with biological targets (e.g., adenosine receptors) using AutoDock Vina. Validate with experimental binding assays .
Q. What strategies resolve contradictions in reported biological activities of this compound derivatives across studies?
Methodological Answer:
- Comparative Assays : Standardize assays (e.g., IC₅₀ measurements) using identical cell lines (e.g., HEK293 for adenosine receptors) and controls .
- Structural Analysis : Correlate activity differences with substituent variations (e.g., diethyl vs. dimethyl groups) via QSAR models.
- Meta-Analysis : Aggregate data from multiple studies (e.g., anti-cancer vs. anti-inflammatory endpoints) to identify consensus mechanisms .
Q. How to design experiments to elucidate the mechanism of action of this compound in biological systems?
Methodological Answer:
- Receptor Binding Assays : Use radiolabeled ligands (e.g., [³H]CGS21680 for A₂A adenosine receptors) to measure competitive inhibition .
- Enzyme Inhibition Studies : Test activity against purine-metabolizing enzymes (e.g., adenosine deaminase) via spectrophotometric monitoring of substrate decay.
- Gene Knockdown : Apply siRNA targeting putative receptors to confirm functional pathways.
Q. Notes on Data Contradictions
- Varied Biological Activities : Discrepancies in anti-cancer vs. anti-inflammatory results may stem from cell-specific uptake or metabolic differences. Use isotopic tracing (e.g., ¹⁴C-labeled compound) to track intracellular distribution .
- Synthetic Yields : Lower yields in scaled-up reactions often relate to inefficient mixing or heat transfer. Optimize via flow chemistry or microwave-assisted synthesis .
Properties
IUPAC Name |
N,N-diethyl-7H-purin-2-amine | |
---|---|---|
Source | PubChem | |
URL | https://pubchem.ncbi.nlm.nih.gov | |
Description | Data deposited in or computed by PubChem | |
InChI |
InChI=1S/C9H13N5/c1-3-14(4-2)9-10-5-7-8(13-9)12-6-11-7/h5-6H,3-4H2,1-2H3,(H,10,11,12,13) | |
Source | PubChem | |
URL | https://pubchem.ncbi.nlm.nih.gov | |
Description | Data deposited in or computed by PubChem | |
InChI Key |
AEKLNDWDOJGCQM-UHFFFAOYSA-N | |
Source | PubChem | |
URL | https://pubchem.ncbi.nlm.nih.gov | |
Description | Data deposited in or computed by PubChem | |
Canonical SMILES |
CCN(CC)C1=NC=C2C(=N1)N=CN2 | |
Source | PubChem | |
URL | https://pubchem.ncbi.nlm.nih.gov | |
Description | Data deposited in or computed by PubChem | |
Molecular Formula |
C9H13N5 | |
Source | PubChem | |
URL | https://pubchem.ncbi.nlm.nih.gov | |
Description | Data deposited in or computed by PubChem | |
Molecular Weight |
191.23 g/mol | |
Source | PubChem | |
URL | https://pubchem.ncbi.nlm.nih.gov | |
Description | Data deposited in or computed by PubChem | |
Retrosynthesis Analysis
AI-Powered Synthesis Planning: Our tool employs the Template_relevance Pistachio, Template_relevance Bkms_metabolic, Template_relevance Pistachio_ringbreaker, Template_relevance Reaxys, Template_relevance Reaxys_biocatalysis model, leveraging a vast database of chemical reactions to predict feasible synthetic routes.
One-Step Synthesis Focus: Specifically designed for one-step synthesis, it provides concise and direct routes for your target compounds, streamlining the synthesis process.
Accurate Predictions: Utilizing the extensive PISTACHIO, BKMS_METABOLIC, PISTACHIO_RINGBREAKER, REAXYS, REAXYS_BIOCATALYSIS database, our tool offers high-accuracy predictions, reflecting the latest in chemical research and data.
Strategy Settings
Precursor scoring | Relevance Heuristic |
---|---|
Min. plausibility | 0.01 |
Model | Template_relevance |
Template Set | Pistachio/Bkms_metabolic/Pistachio_ringbreaker/Reaxys/Reaxys_biocatalysis |
Top-N result to add to graph | 6 |
Feasible Synthetic Routes
Disclaimer and Information on In-Vitro Research Products
Please be aware that all articles and product information presented on BenchChem are intended solely for informational purposes. The products available for purchase on BenchChem are specifically designed for in-vitro studies, which are conducted outside of living organisms. In-vitro studies, derived from the Latin term "in glass," involve experiments performed in controlled laboratory settings using cells or tissues. It is important to note that these products are not categorized as medicines or drugs, and they have not received approval from the FDA for the prevention, treatment, or cure of any medical condition, ailment, or disease. We must emphasize that any form of bodily introduction of these products into humans or animals is strictly prohibited by law. It is essential to adhere to these guidelines to ensure compliance with legal and ethical standards in research and experimentation.