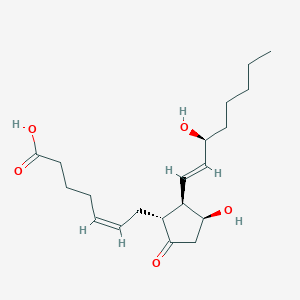
11beta-PGE2
Overview
Description
11β-PGE2 is the C-11 epimer of PGE2. It is a moderate inhibitor of PGE2 binding to rat hypothalamic membranes with a Ki value of 53 nM. 11β-PGE2 also stimulates bone resorption in rats at concentrations of 10 to 1,000 nM which is similar to PGE2.2 11β-PGE2 inhibits PGE2 binding to the prostaglandin transporter protein with a Ki of 56 nM.
11beta-Pge2, also known as 11b-pge2, belongs to the class of organic compounds known as prostaglandins and related compounds. These are unsaturated carboxylic acids consisting of a 20 carbon skeleton that also contains a five member ring, and are based upon the fatty acid arachidonic acid. Thus, this compound is considered to be an eicosanoid lipid molecule. This compound is considered to be a practically insoluble (in water) and relatively neutral molecule. This compound has been detected in multiple biofluids, such as blood and urine. Within the cell, this compound is primarily located in the membrane (predicted from logP) and cytoplasm.
11b-PGE2 is a prostanoid.
Mechanism of Action
Target of Action
11beta-Prostaglandin E2 (11beta-PGE2) is a variant of Prostaglandin E2 (PGE2), a naturally occurring prostaglandin . The primary targets of this compound are the prostaglandin E2 receptors, namely EP1, EP2, EP3, and EP4 . These receptors are G protein-coupled receptors with distinct signaling properties . This compound may also interact with the prostaglandin transporter protein .
Mode of Action
This compound acts as a moderate inhibitor of PGE2 binding to rat hypothalamic membranes . It interacts with its targets, the PGE2 receptors, leading to various downstream effects. The interaction of this compound with these receptors can influence blood pressure both positively and negatively . For instance, centrally administered PGE2 induces hypertension, whereas systemic administration of PGE2 produces a hypotensive effect .
Biochemical Pathways
The action of this compound affects several biochemical pathways. It is involved in the prostanoid pathway, where it is converted from arachidonic acid (AA) by a series of enzymatic reactions involving phospholipases, cyclooxygenase (COX), and distinct terminal synthases . The conversion of AA to an unstable endoperoxide intermediate PGH2 by COX is followed by the isomerization of PGH2 to bioactive prostanoids, including PGE2 .
Pharmacokinetics
The synthetic pge2 dinoprostone, a related compound, has a plasma half-life of approximately 25–5 minutes after vaginal administration, with most metabolites being excreted in the urine .
Result of Action
The action of this compound results in various molecular and cellular effects. It may act to stimulate bone resorption in rats . In the context of blood pressure regulation, the interaction of this compound with its receptors can lead to hypertension or hypotension, depending on the route of administration .
Action Environment
The action, efficacy, and stability of this compound can be influenced by environmental factors. For instance, the intestinal microenvironment, which is rich in PGE2, can drive mitochondrial depolarization in CD8+ T cells . This leads to the engagement of autophagy to clear depolarized mitochondria and enhances glutathione synthesis to scavenge reactive oxygen species (ROS) that result from mitochondrial depolarization .
Biochemical Analysis
Biochemical Properties
11beta-Prostaglandin E2 interacts with various enzymes, proteins, and other biomolecules. It is a moderate inhibitor of PGE2 binding to rat hypothalamic membranes . It may also stimulate bone resorption in rats and inhibit PGE2 binding to the prostaglandin transporter protein .
Cellular Effects
11beta-Prostaglandin E2 has profound effects on various types of cells and cellular processes. It influences cell function by modulating inflammation and the immune system by regulating the expression/concentration of cytokines . It also plays a role in the immune system, with regards to immunosuppression, inhibition of nitrogen oxide (NO) production, inhibition of interferon (IFN) and apoptotic pathways, and inhibition of viral receptor expression .
Molecular Mechanism
At the molecular level, 11beta-Prostaglandin E2 exerts its effects through various mechanisms. It is involved in the synthesis of the prostanoids (prostaglandins, prostacyclins, and thromboxanes) and is implicated in normal development, tissue homeostasis, inflammation, and cancer progression . It also plays a role in the production of PGE2 involved in both tissue homeostasis and disease .
Metabolic Pathways
11beta-Prostaglandin E2 is involved in the prostanoid metabolic pathway. Prostanoids are signaling molecules derived from arachidonic acid (AA). They are classified by their primary physiological functions: prostacyclins that function in the resolution of inflammation, thromboxanes that function in the related roles of platelet aggregation and vasoconstriction, and prostaglandins that are involved in pro-inflammatory processes .
Subcellular Localization
It is known that PGE2 is synthesized as a Golgi membrane-associated protein, and spontaneous cleavage of the N-terminal hydrophobic domain leads to the formation of a truncated mature protein that is distributed in the cytosol with a trend to be enriched in the perinuclear region .
Properties
IUPAC Name |
(Z)-7-[(1R,2R,3S)-3-hydroxy-2-[(E,3S)-3-hydroxyoct-1-enyl]-5-oxocyclopentyl]hept-5-enoic acid | |
---|---|---|
Source | PubChem | |
URL | https://pubchem.ncbi.nlm.nih.gov | |
Description | Data deposited in or computed by PubChem | |
InChI |
InChI=1S/C20H32O5/c1-2-3-6-9-15(21)12-13-17-16(18(22)14-19(17)23)10-7-4-5-8-11-20(24)25/h4,7,12-13,15-17,19,21,23H,2-3,5-6,8-11,14H2,1H3,(H,24,25)/b7-4-,13-12+/t15-,16+,17+,19-/m0/s1 | |
Source | PubChem | |
URL | https://pubchem.ncbi.nlm.nih.gov | |
Description | Data deposited in or computed by PubChem | |
InChI Key |
XEYBRNLFEZDVAW-YUOXZBOXSA-N | |
Source | PubChem | |
URL | https://pubchem.ncbi.nlm.nih.gov | |
Description | Data deposited in or computed by PubChem | |
Canonical SMILES |
CCCCCC(C=CC1C(CC(=O)C1CC=CCCCC(=O)O)O)O | |
Source | PubChem | |
URL | https://pubchem.ncbi.nlm.nih.gov | |
Description | Data deposited in or computed by PubChem | |
Isomeric SMILES |
CCCCC[C@@H](/C=C/[C@H]1[C@H](CC(=O)[C@@H]1C/C=C\CCCC(=O)O)O)O | |
Source | PubChem | |
URL | https://pubchem.ncbi.nlm.nih.gov | |
Description | Data deposited in or computed by PubChem | |
Molecular Formula |
C20H32O5 | |
Source | PubChem | |
URL | https://pubchem.ncbi.nlm.nih.gov | |
Description | Data deposited in or computed by PubChem | |
DSSTOX Substance ID |
DTXSID301017289 | |
Record name | 11β-Prostaglandin E2 | |
Source | EPA DSSTox | |
URL | https://comptox.epa.gov/dashboard/DTXSID301017289 | |
Description | DSSTox provides a high quality public chemistry resource for supporting improved predictive toxicology. | |
Molecular Weight |
352.5 g/mol | |
Source | PubChem | |
URL | https://pubchem.ncbi.nlm.nih.gov | |
Description | Data deposited in or computed by PubChem | |
CAS No. |
38310-90-6 | |
Record name | 11β-Prostaglandin E2 | |
Source | EPA DSSTox | |
URL | https://comptox.epa.gov/dashboard/DTXSID301017289 | |
Description | DSSTox provides a high quality public chemistry resource for supporting improved predictive toxicology. | |
Retrosynthesis Analysis
AI-Powered Synthesis Planning: Our tool employs the Template_relevance Pistachio, Template_relevance Bkms_metabolic, Template_relevance Pistachio_ringbreaker, Template_relevance Reaxys, Template_relevance Reaxys_biocatalysis model, leveraging a vast database of chemical reactions to predict feasible synthetic routes.
One-Step Synthesis Focus: Specifically designed for one-step synthesis, it provides concise and direct routes for your target compounds, streamlining the synthesis process.
Accurate Predictions: Utilizing the extensive PISTACHIO, BKMS_METABOLIC, PISTACHIO_RINGBREAKER, REAXYS, REAXYS_BIOCATALYSIS database, our tool offers high-accuracy predictions, reflecting the latest in chemical research and data.
Strategy Settings
Precursor scoring | Relevance Heuristic |
---|---|
Min. plausibility | 0.01 |
Model | Template_relevance |
Template Set | Pistachio/Bkms_metabolic/Pistachio_ringbreaker/Reaxys/Reaxys_biocatalysis |
Top-N result to add to graph | 6 |
Feasible Synthetic Routes
Disclaimer and Information on In-Vitro Research Products
Please be aware that all articles and product information presented on BenchChem are intended solely for informational purposes. The products available for purchase on BenchChem are specifically designed for in-vitro studies, which are conducted outside of living organisms. In-vitro studies, derived from the Latin term "in glass," involve experiments performed in controlled laboratory settings using cells or tissues. It is important to note that these products are not categorized as medicines or drugs, and they have not received approval from the FDA for the prevention, treatment, or cure of any medical condition, ailment, or disease. We must emphasize that any form of bodily introduction of these products into humans or animals is strictly prohibited by law. It is essential to adhere to these guidelines to ensure compliance with legal and ethical standards in research and experimentation.