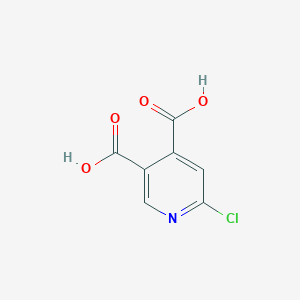
6-chloropyridine-3,4-dicarboxylic Acid
Overview
Description
6-Chloropyridine-3,4-dicarboxylic acid is a chemical compound with the CAS Number: 243835-70-3 . It has a molecular weight of 201.57 and its IUPAC name is 6-chloro-3,4-pyridinedicarboxylic acid .
Molecular Structure Analysis
The InChI code for this compound is 1S/C7H4ClNO4/c8-5-1-3 (6 (10)11)4 (2-9-5)7 (12)13/h1-2H, (H,10,11) (H,12,13) . This indicates the presence of a pyridine ring with chlorine and carboxylic acid groups attached.Physical And Chemical Properties Analysis
This compound has a molecular weight of 201.57 . Other physical and chemical properties such as melting point, boiling point, and density are not explicitly mentioned in the available resources.Scientific Research Applications
Synthesis and Structural Characterization
- Synthetic Process Improvement: The synthesis of chloropyridine dicarboxylic acids, closely related to 6-chloropyridine-3,4-dicarboxylic acid, involves practical and industrially scalable processes. Xiao (2011) improved the synthetic process of 4-chloropyridine-2,6-dicarboxylic acid, achieving a 38% overall yield, demonstrating its industrial feasibility (Xiao, 2011).
Complex Synthesis and Properties
- Complex Formation with Metals: Wang et al. (2022) synthesized complexes using 4-chloropyridine-2,6-dicarboxylic acid as a ligand. These complexes, including nickel and copper-based structures, exhibit unique properties like three-dimensional network structures and hydrogen bonding interactions (Wang et al., 2022).
Applications in Organic Synthesis
- Intermediate for Pharmaceutical Synthesis: Xian-hua (2008) reported the synthesis of 6-Benzylpyrrolo-[3,4-b] pyridine, a key intermediate for Moxifloxacin, from pyridine-2,3-dicarboxylic acid, indicating the potential of chloropyridine dicarboxylic acids in pharmaceutical synthesis (Xian-hua, 2008).
Analytical and Luminescence Studies
- Electroreduction Studies: Corredor and Mellado (2006) studied the electroreduction of 6-chloro-2-pyridinecarboxylic acid on mercury electrodes, providing insights into its electrochemical behavior, crucial for analytical applications (Corredor & Mellado, 2006).
- Luminescent Properties of Complexes: Rui-ren et al. (2006) synthesized Eu(III) and Tb(III) complexes using derivatives of pyridine-2,6-dicarboxylic acid, exploring their potential in fluorescence-based applications like time-resolved fluoroimmunoassays (Rui-ren et al., 2006).
Environmental and Biological Applications
- Mercury(II) Preconcentration: Zhang et al. (2010) utilized 2,6-pyridine dicarboxylic acid functionalized silica for the extraction and preconcentration of mercury(II) from environmental and biological samples, showcasing its utility in environmental analysis (Zhang et al., 2010).
Safety and Hazards
While specific safety and hazard information for 6-chloropyridine-3,4-dicarboxylic acid is not available, it’s important to handle all chemical compounds with care. Personal protective equipment such as gloves, safety glasses, and protective masks are generally recommended when handling chemical compounds .
Mechanism of Action
Mode of Action
It is known that the compound can undergo reactions at the benzylic position, which includes free radical bromination, nucleophilic substitution, and oxidation .
Biochemical Pathways
It is known that the compound is a degradation product of neonicotinoid insecticides imidacloprid and acetamiprid .
Action Environment
Environmental factors can influence the action, efficacy, and stability of 6-chloropyridine-3,4-dicarboxylic Acid. For instance, it is known to appear in different environmental matrices as a degradation product of neonicotinoid insecticides . .
properties
IUPAC Name |
6-chloropyridine-3,4-dicarboxylic acid | |
---|---|---|
Source | PubChem | |
URL | https://pubchem.ncbi.nlm.nih.gov | |
Description | Data deposited in or computed by PubChem | |
InChI |
InChI=1S/C7H4ClNO4/c8-5-1-3(6(10)11)4(2-9-5)7(12)13/h1-2H,(H,10,11)(H,12,13) | |
Source | PubChem | |
URL | https://pubchem.ncbi.nlm.nih.gov | |
Description | Data deposited in or computed by PubChem | |
InChI Key |
LVQPMCFUDXELIS-UHFFFAOYSA-N | |
Source | PubChem | |
URL | https://pubchem.ncbi.nlm.nih.gov | |
Description | Data deposited in or computed by PubChem | |
Canonical SMILES |
C1=C(C(=CN=C1Cl)C(=O)O)C(=O)O | |
Source | PubChem | |
URL | https://pubchem.ncbi.nlm.nih.gov | |
Description | Data deposited in or computed by PubChem | |
Molecular Formula |
C7H4ClNO4 | |
Source | PubChem | |
URL | https://pubchem.ncbi.nlm.nih.gov | |
Description | Data deposited in or computed by PubChem | |
DSSTOX Substance ID |
DTXSID30376260 | |
Record name | 6-chloropyridine-3,4-dicarboxylic Acid | |
Source | EPA DSSTox | |
URL | https://comptox.epa.gov/dashboard/DTXSID30376260 | |
Description | DSSTox provides a high quality public chemistry resource for supporting improved predictive toxicology. | |
Molecular Weight |
201.56 g/mol | |
Source | PubChem | |
URL | https://pubchem.ncbi.nlm.nih.gov | |
Description | Data deposited in or computed by PubChem | |
CAS RN |
243835-70-3 | |
Record name | 6-chloropyridine-3,4-dicarboxylic Acid | |
Source | EPA DSSTox | |
URL | https://comptox.epa.gov/dashboard/DTXSID30376260 | |
Description | DSSTox provides a high quality public chemistry resource for supporting improved predictive toxicology. | |
Retrosynthesis Analysis
AI-Powered Synthesis Planning: Our tool employs the Template_relevance Pistachio, Template_relevance Bkms_metabolic, Template_relevance Pistachio_ringbreaker, Template_relevance Reaxys, Template_relevance Reaxys_biocatalysis model, leveraging a vast database of chemical reactions to predict feasible synthetic routes.
One-Step Synthesis Focus: Specifically designed for one-step synthesis, it provides concise and direct routes for your target compounds, streamlining the synthesis process.
Accurate Predictions: Utilizing the extensive PISTACHIO, BKMS_METABOLIC, PISTACHIO_RINGBREAKER, REAXYS, REAXYS_BIOCATALYSIS database, our tool offers high-accuracy predictions, reflecting the latest in chemical research and data.
Strategy Settings
Precursor scoring | Relevance Heuristic |
---|---|
Min. plausibility | 0.01 |
Model | Template_relevance |
Template Set | Pistachio/Bkms_metabolic/Pistachio_ringbreaker/Reaxys/Reaxys_biocatalysis |
Top-N result to add to graph | 6 |
Feasible Synthetic Routes
Disclaimer and Information on In-Vitro Research Products
Please be aware that all articles and product information presented on BenchChem are intended solely for informational purposes. The products available for purchase on BenchChem are specifically designed for in-vitro studies, which are conducted outside of living organisms. In-vitro studies, derived from the Latin term "in glass," involve experiments performed in controlled laboratory settings using cells or tissues. It is important to note that these products are not categorized as medicines or drugs, and they have not received approval from the FDA for the prevention, treatment, or cure of any medical condition, ailment, or disease. We must emphasize that any form of bodily introduction of these products into humans or animals is strictly prohibited by law. It is essential to adhere to these guidelines to ensure compliance with legal and ethical standards in research and experimentation.