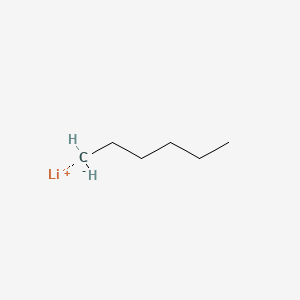
n-Hexyllithium
Overview
Description
n-Hexyllithium, with the chemical formula C₆H₁₃Li, is an organolithium compound widely used in organic synthesis. It is typically encountered as a colorless or pale yellow solution in hexanes. This compound is highly sensitive to air and can ignite when exposed to water. This compound is primarily used as a strong base or as a lithiation reagent in various chemical reactions .
Mechanism of Action
Target of Action
n-Hexyllithium is an organolithium compound used in organic synthesis as a strong base or as a lithiation reagent . It primarily targets unsaturated functional groups, for example, 1,2-additions to carbonyl compounds or CN double and triple bonds .
Mode of Action
this compound interacts with its targets by serving as carbanion equivalents and is used directly or indirectly for various C-C cross-coupling reactions . It is involved in deprotonation reactions and bromine/lithium exchange reactions . As a base, this compound generates n-hexane as a byproduct .
Biochemical Pathways
The primary biochemical pathways affected by this compound are those involving deprotonation reactions and halogen/metal exchange reactions . These reactions are crucial for the production of organozinc or organocopper compounds and for direct transition metal-catalyzed C-C coupling reactions .
Pharmacokinetics
It is usually encountered as a colorless or pale yellow solution in hexanes . Such solutions are highly sensitive to air and can ignite when treated with water .
Result of Action
The molecular and cellular effects of this compound’s action primarily involve the formation of new carbon-carbon bonds through various reactions, including deprotonation and bromine/lithium exchange reactions . This makes it a valuable tool in organic synthesis .
Action Environment
The action, efficacy, and stability of this compound are significantly influenced by environmental factors. It is highly sensitive to air and can ignite when treated with water . Therefore, it is typically used in controlled environments that minimize exposure to air and moisture .
Biochemical Analysis
Biochemical Properties
n-Hexyllithium is a strong base and serves as a lithiation reagent . It plays a crucial role in various biochemical reactions, particularly in the deprotonation and bromine/lithium exchange reactions
Molecular Mechanism
The molecular mechanism of this compound primarily involves its function as a strong base and a lithiation reagent . It participates in deprotonation and bromine/lithium exchange reactions
Temporal Effects in Laboratory Settings
This compound is used in continuous processes carried out in micro and mesoreactors . The observed reactions could be carried out under more convenient conditions, at higher temperatures compared to the batch mode
Preparation Methods
Synthetic Routes and Reaction Conditions: n-Hexyllithium is commonly prepared by the reaction of hexyl halides (such as hexyl chloride or hexyl bromide) with lithium metal. The reaction is typically carried out in an inert atmosphere, such as nitrogen or argon, to prevent the compound from reacting with moisture or oxygen. The general reaction is as follows:
C6H13X+2Li→C6H13Li+LiX
where X represents a halogen (Cl or Br).
Industrial Production Methods: In industrial settings, this compound is produced in large quantities using continuous flow processes. These processes involve the use of micro and mesoreactors, which offer improved safety and efficiency compared to traditional batch processes. The continuous flow method allows for better control over reaction conditions and reduces the risk of hazardous incidents .
Chemical Reactions Analysis
Types of Reactions: n-Hexyllithium undergoes various types of reactions, including:
Deprotonation: It acts as a strong base and can deprotonate a wide range of acidic compounds, generating n-hexane as a byproduct.
Halogen-Metal Exchange: It can react with halogenated compounds to replace the halogen with a lithium atom.
Addition Reactions: It can add to unsaturated functional groups, such as carbonyl compounds, to form new carbon-carbon bonds.
Common Reagents and Conditions:
Deprotonation: Typically carried out in hydrocarbon solvents like hexane or tetrahydrofuran (THF) at low temperatures.
Halogen-Metal Exchange: Often performed with halogenated compounds like alkyl halides or aryl halides in the presence of an inert atmosphere.
Addition Reactions: Conducted in solvents like THF or diethyl ether, often at low temperatures to control the reactivity.
Major Products:
Deprotonation: Produces n-hexane as a byproduct.
Halogen-Metal Exchange: Forms organolithium compounds.
Addition Reactions: Generates new carbon-carbon bonds, leading to various organic products
Scientific Research Applications
n-Hexyllithium has a wide range of applications in scientific research, including:
Organic Synthesis: Used as a strong base and lithiation reagent in the synthesis of complex organic molecules.
Pharmaceuticals: Employed in the production of pharmaceutical intermediates and active pharmaceutical ingredients.
Agrochemicals: Utilized in the synthesis of agrochemical products, such as pesticides and herbicides.
Material Science: Involved in the preparation of advanced materials, including polymers and nanomaterials.
Comparison with Similar Compounds
n-Butyllithium: Similar in reactivity but generates butane as a byproduct instead of n-hexane.
Methyllithium: More reactive due to the smaller size of the methyl group.
tert-Butyllithium: Highly reactive and often used in sterically hindered environments.
Uniqueness of n-Hexyllithium: this compound is unique in that it generates n-hexane as a byproduct, which is a liquid and less hazardous compared to the gaseous butane produced by n-butyllithium. Additionally, this compound is slightly less reactive, making it more manageable in certain industrial applications .
Properties
IUPAC Name |
lithium;hexane | |
---|---|---|
Source | PubChem | |
URL | https://pubchem.ncbi.nlm.nih.gov | |
Description | Data deposited in or computed by PubChem | |
InChI |
InChI=1S/C6H13.Li/c1-3-5-6-4-2;/h1,3-6H2,2H3;/q-1;+1 | |
Source | PubChem | |
URL | https://pubchem.ncbi.nlm.nih.gov | |
Description | Data deposited in or computed by PubChem | |
InChI Key |
CETVQRFGPOGIQJ-UHFFFAOYSA-N | |
Source | PubChem | |
URL | https://pubchem.ncbi.nlm.nih.gov | |
Description | Data deposited in or computed by PubChem | |
Canonical SMILES |
[Li+].CCCCC[CH2-] | |
Source | PubChem | |
URL | https://pubchem.ncbi.nlm.nih.gov | |
Description | Data deposited in or computed by PubChem | |
Molecular Formula |
C6H13Li | |
Source | PubChem | |
URL | https://pubchem.ncbi.nlm.nih.gov | |
Description | Data deposited in or computed by PubChem | |
DSSTOX Substance ID |
DTXSID0074899 | |
Record name | Lithium, hexyl- | |
Source | EPA DSSTox | |
URL | https://comptox.epa.gov/dashboard/DTXSID0074899 | |
Description | DSSTox provides a high quality public chemistry resource for supporting improved predictive toxicology. | |
Molecular Weight |
92.1 g/mol | |
Source | PubChem | |
URL | https://pubchem.ncbi.nlm.nih.gov | |
Description | Data deposited in or computed by PubChem | |
CAS No. |
21369-64-2 | |
Record name | Lithium, hexyl- | |
Source | ChemIDplus | |
URL | https://pubchem.ncbi.nlm.nih.gov/substance/?source=chemidplus&sourceid=0021369642 | |
Description | ChemIDplus is a free, web search system that provides access to the structure and nomenclature authority files used for the identification of chemical substances cited in National Library of Medicine (NLM) databases, including the TOXNET system. | |
Record name | Lithium, hexyl- | |
Source | EPA Chemicals under the TSCA | |
URL | https://www.epa.gov/chemicals-under-tsca | |
Description | EPA Chemicals under the Toxic Substances Control Act (TSCA) collection contains information on chemicals and their regulations under TSCA, including non-confidential content from the TSCA Chemical Substance Inventory and Chemical Data Reporting. | |
Record name | Lithium, hexyl- | |
Source | EPA DSSTox | |
URL | https://comptox.epa.gov/dashboard/DTXSID0074899 | |
Description | DSSTox provides a high quality public chemistry resource for supporting improved predictive toxicology. | |
Record name | N-hexyllithium | |
Source | European Chemicals Agency (ECHA) | |
URL | https://echa.europa.eu/substance-information/-/substanceinfo/100.100.655 | |
Description | The European Chemicals Agency (ECHA) is an agency of the European Union which is the driving force among regulatory authorities in implementing the EU's groundbreaking chemicals legislation for the benefit of human health and the environment as well as for innovation and competitiveness. | |
Explanation | Use of the information, documents and data from the ECHA website is subject to the terms and conditions of this Legal Notice, and subject to other binding limitations provided for under applicable law, the information, documents and data made available on the ECHA website may be reproduced, distributed and/or used, totally or in part, for non-commercial purposes provided that ECHA is acknowledged as the source: "Source: European Chemicals Agency, http://echa.europa.eu/". Such acknowledgement must be included in each copy of the material. ECHA permits and encourages organisations and individuals to create links to the ECHA website under the following cumulative conditions: Links can only be made to webpages that provide a link to the Legal Notice page. | |
Record name | N-HEXYLLITHIUM | |
Source | European Chemicals Agency (ECHA) | |
URL | https://echa.europa.eu/information-on-chemicals | |
Description | The European Chemicals Agency (ECHA) is an agency of the European Union which is the driving force among regulatory authorities in implementing the EU's groundbreaking chemicals legislation for the benefit of human health and the environment as well as for innovation and competitiveness. | |
Explanation | Use of the information, documents and data from the ECHA website is subject to the terms and conditions of this Legal Notice, and subject to other binding limitations provided for under applicable law, the information, documents and data made available on the ECHA website may be reproduced, distributed and/or used, totally or in part, for non-commercial purposes provided that ECHA is acknowledged as the source: "Source: European Chemicals Agency, http://echa.europa.eu/". Such acknowledgement must be included in each copy of the material. ECHA permits and encourages organisations and individuals to create links to the ECHA website under the following cumulative conditions: Links can only be made to webpages that provide a link to the Legal Notice page. | |
Retrosynthesis Analysis
AI-Powered Synthesis Planning: Our tool employs the Template_relevance Pistachio, Template_relevance Bkms_metabolic, Template_relevance Pistachio_ringbreaker, Template_relevance Reaxys, Template_relevance Reaxys_biocatalysis model, leveraging a vast database of chemical reactions to predict feasible synthetic routes.
One-Step Synthesis Focus: Specifically designed for one-step synthesis, it provides concise and direct routes for your target compounds, streamlining the synthesis process.
Accurate Predictions: Utilizing the extensive PISTACHIO, BKMS_METABOLIC, PISTACHIO_RINGBREAKER, REAXYS, REAXYS_BIOCATALYSIS database, our tool offers high-accuracy predictions, reflecting the latest in chemical research and data.
Strategy Settings
Precursor scoring | Relevance Heuristic |
---|---|
Min. plausibility | 0.01 |
Model | Template_relevance |
Template Set | Pistachio/Bkms_metabolic/Pistachio_ringbreaker/Reaxys/Reaxys_biocatalysis |
Top-N result to add to graph | 6 |
Feasible Synthetic Routes
Q1: What is the primary application of n-hexyllithium in organic chemistry?
A1: this compound is primarily used as a strong base and a nucleophile in organic synthesis. [, , , , ] It can deprotonate a wide range of organic compounds, generating reactive carbanion intermediates for further transformations. [, , ] For instance, it can facilitate ortho-lithiation of arenes containing directing groups like azetidine, allowing for regioselective functionalization. []
Q2: How does this compound interact with nickel(II) acetylacetonate?
A2: this compound acts as a reducing agent in its reaction with nickel(II) acetylacetonate. [] Complete reduction of one mole of nickel(II) acetylacetonate requires four moles of this compound, producing n-hexane and isomeric n-hexenes as byproducts. [] The presence of triphenylphosphane (PPh3) alters this reaction, requiring at least five moles of this compound and leading to the formation of biphenyl. []
Q3: What are the structural characteristics of this compound?
A3: this compound has the molecular formula C6H13Li and a molecular weight of 98.14 g/mol. [] While spectroscopic data like NMR can provide insights into its structure and behavior in solution, detailed characterization often relies on techniques like 13C and 6Li NMR spectroscopy. []
Q4: How does the structure of the alkyl group in alkyllithium compounds influence their aggregation behavior?
A4: The structure of the alkyl group significantly affects the aggregation state of alkyllithium compounds in solution. [] Studies employing 13C and 6Li NMR spectroscopy show that branching at the alpha or beta carbon of the alkyl group leads to observable 13C-6Li coupling, indicating the presence of specific aggregates and their potential fluxional behavior. []
Q5: What are the safety considerations when working with this compound?
A5: this compound is a highly reactive compound, sensitive to air and moisture. [, , ] It reacts violently with water, generating flammable hexane and corrosive lithium hydroxide. Therefore, handling this reagent requires strict inert atmosphere techniques, such as working under nitrogen or argon, and using dry solvents. [, , ]
Q6: How does the choice of solvent affect the reactivity of this compound?
A6: The solvent plays a crucial role in the reactivity of this compound. [, , ] Polar solvents like tetrahydrofuran (THF) can solvate the lithium cation more effectively, increasing the reactivity of the alkyllithium species. [, ] For instance, 2-MeTHF has been highlighted as a greener alternative to traditional solvents when using this compound for regioselective functionalization. []
Q7: What are the advantages of using this compound over other alkyllithium reagents like n-butyllithium?
A7: this compound is often considered a safer alternative to n-butyllithium in certain reactions. [] While both reagents share similar reactivity profiles, this compound exhibits a higher flash point, making it less prone to ignition upon accidental exposure to air. [] This safety advantage makes this compound a preferable choice in some synthetic applications.
Q8: How is this compound used in polymer chemistry?
A8: this compound, particularly its derivative 1,1-diphenyl-n-hexyllithium, acts as an initiator in anionic polymerization reactions. [, , ] These initiators can trigger the polymerization of monomers like methyl methacrylate and methacrylonitrile, leading to the formation of well-defined polymers with controlled molecular weight and tacticity. [, , ]
Q9: Has computational chemistry been applied to study this compound?
A9: While the provided abstracts don't delve into specific computational studies on this compound, computational methods like density functional theory (DFT) are frequently employed to investigate the structure, bonding, and reactivity of organolithium compounds. [] These calculations can provide valuable insights into the reaction mechanisms involving this compound and help predict its behavior in various chemical environments.
Disclaimer and Information on In-Vitro Research Products
Please be aware that all articles and product information presented on BenchChem are intended solely for informational purposes. The products available for purchase on BenchChem are specifically designed for in-vitro studies, which are conducted outside of living organisms. In-vitro studies, derived from the Latin term "in glass," involve experiments performed in controlled laboratory settings using cells or tissues. It is important to note that these products are not categorized as medicines or drugs, and they have not received approval from the FDA for the prevention, treatment, or cure of any medical condition, ailment, or disease. We must emphasize that any form of bodily introduction of these products into humans or animals is strictly prohibited by law. It is essential to adhere to these guidelines to ensure compliance with legal and ethical standards in research and experimentation.