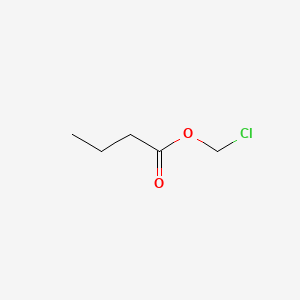
Chloromethyl butyrate
Overview
Description
Chloromethyl butyrate (C₅H₉ClO₂), also known as chloromethyl butanoate or butyric acid chloromethyl ester, is an organic ester compound characterized by a chloromethyl group attached to a butyrate moiety. It is primarily utilized in chemical synthesis, pharmaceutical intermediates, and biochemical research due to its reactivity as an acylating agent . Key identifiers include CAS numbers and synonyms such as propylcarbonyloxymethyl chloride and chloromethyl n-butyrate .
Preparation Methods
Preparation Methods
Synthesis from γ-Butyrolactone, Methanol, and Phosphorus Trichloride
One well-documented and industrially relevant preparation method involves the reaction of γ-butyrolactone with methanol in the presence of an acidic catalyst and phosphorus trichloride (PCl3). This method is notable for its mild reaction conditions, simplicity, and environmentally friendly raw materials.
$$
\gamma\text{-butyrolactone} + \text{methanol} + \text{PCl}_3 \xrightarrow[\text{acidic catalyst}]{30-60^\circ C} \text{methyl 4-chlorobutyrate} + \text{by-products}
$$
- Acidic catalyst: Zinc chloride (ZnCl2) at 1–5 mol% relative to γ-butyrolactone
- Molar ratios: γ-butyrolactone to methanol = 1:2 to 1:5; γ-butyrolactone to PCl3 = 1:0.35 to 1:1
- Temperature: 30–60 °C
- Pressure: Atmospheric pressure
- Stirring speed: 1000–2000 rpm
- Reaction time: Dropwise addition of PCl3 over 30 minutes, followed by 0.5–2 hours of post-reaction at constant temperature
- The reaction mixture is subjected to vacuum distillation at 25 mm Hg, collecting fractions at 80–85 °C to isolate the product.
Representative Experimental Data:
Example | γ-Butyrolactone (g, mol) | Methanol (mL, mol) | ZnCl2 (g, mol) | PCl3 (g, mol) | Reaction Temp (°C) | Yield (%) | Purity (%) |
---|---|---|---|---|---|---|---|
1 | 43 (0.5) | 40 (1.5) | 1.36 (0.01) | 34.3 (0.25) | 50 | 94.5 | 99.1 |
2 | 43 (0.5) | 60 (1.0) | 1.36 (0.01) | 34.3 (0.25) | 50 | 85.5 | 99.0 |
This method offers advantages such as mild reaction conditions, simple operation, low waste generation, and straightforward purification.
Esterification of Chloroformic Acid with n-Butanol
Another synthetic route involves the esterification of chloroformic acid with n-butanol under acidic catalysis. This method is commonly used in industrial settings where large-scale production is required.
$$
\text{Chloroformic acid} + \text{n-butanol} \xrightarrow[\text{acid catalyst}]{} \text{chloromethyl butyrate} + \text{water}
$$
- Acidic catalyst facilitates ester bond formation.
- Reaction conditions are optimized for high yield and purity.
- Industrial production often employs continuous flow reactors to maintain consistent reaction parameters.
This method is less detailed in available literature but is recognized for scalability and efficiency in industrial manufacturing.
Comparative Analysis of Preparation Methods
Method | Key Reagents | Conditions | Yield (%) | Purity (%) | Advantages | Limitations |
---|---|---|---|---|---|---|
γ-Butyrolactone + Methanol + PCl3 (ZnCl2 catalyst) | γ-butyrolactone, methanol, PCl3, ZnCl2 | 30–60 °C, atmospheric pressure | Up to 94.5 | ~99 | Mild conditions, simple process, eco-friendly | Requires careful handling of PCl3 |
Chloroformic acid + n-butanol (acid catalyst) | Chloroformic acid, n-butanol | Acidic catalysis, industrial scale | High | High | Scalable, continuous flow compatible | Less detailed procedural data |
Research Findings and Notes
- The zinc chloride-catalyzed method using γ-butyrolactone and phosphorus trichloride is well-documented in patent literature, showing high yields and purity with relatively mild conditions and simple post-reaction workup.
- Industrial methods favor esterification of chloroformic acid with n-butanol, optimized for large-scale production with continuous reactors to maintain product consistency.
- This compound’s reactivity as an alkylating agent in nucleophilic substitution reactions underpins its importance as a synthetic intermediate.
- Handling of phosphorus trichloride and chloroformic acid requires strict safety measures due to their corrosive and reactive nature.
- Reaction parameters such as molar ratios, temperature, and catalyst loading significantly influence yield and purity.
Chemical Reactions Analysis
Types of Reactions: Chloromethyl butyrate undergoes various chemical reactions, including:
Substitution Reactions: It can participate in nucleophilic substitution reactions where the chlorine atom is replaced by other nucleophiles.
Esterification Reactions: It can be used to form esters with different alcohols.
Common Reagents and Conditions:
Nucleophilic Substitution: Reagents such as potassium carbonate (K2CO3) in dimethylformamide (DMF) are commonly used.
Esterification: Acidic catalysts are used to facilitate the esterification process.
Major Products:
Substituted Esters: These are formed when this compound reacts with nucleophiles.
Alcohol Derivatives: Formed through esterification reactions with various alcohols.
Scientific Research Applications
Cardiac Metabolism Research
CMB has been utilized as a hyperpolarized substrate in dynamic nuclear polarization-enhanced magnetic resonance spectroscopy. This application allows researchers to study short-chain fatty acid metabolism in the heart, providing insights into cardiac metabolic pathways and potential therapeutic targets for heart diseases (Ball et al., 2013).
Pharmaceutical Synthesis
In pharmaceutical manufacturing, CMB is crucial for synthesizing clevidipine butyrate. Raman spectroscopy has been employed to monitor its transformation in real-time, enhancing the efficiency and accuracy of pharmaceutical synthesis processes (Liu et al., 2017).
Gut Health and Disease Prevention
Research indicates that butyrate derivatives, including those from CMB, are vital for maintaining gut health. They serve as primary nutrients for colonocytes and regulate gene expression, immune modulation, and oxidative stress reduction, making them potential therapeutic agents for intestinal diseases (Bedford & Gong, 2017).
Epigenetic Regulation
CMB-derived butyrate acts as a histone deacetylase inhibitor, influencing gene expression. This property is significant in developing therapeutic strategies for genetic/metabolic conditions and neurological disorders (Canani et al., 2012).
Environmental Applications
CMB's derivative poly-3-hydroxy-butyrate (PHB) has been applied in bioremediation efforts to treat contaminated aquifers by serving as an electron donor for degrading chlorinated hydrocarbons (Pierro et al., 2017).
Metabolic Disorders
Butyrate derived from CMB plays a role in regulating insulin sensitivity and energy metabolism, indicating its potential therapeutic applications in dietary-induced obesity and diabetes (Gao et al., 2009).
Non-alcoholic Steatohepatitis (NASH)
Studies have shown that butyrate can modify the gut microbiome and metabolome, providing protective effects against NASH and related metabolic disorders (Ye et al., 2018).
Data Table: Applications of Chloromethyl Butyrate
Application Area | Specific Use Case | Key Findings/Outcomes |
---|---|---|
Cardiac Research | Hyperpolarized substrate for MRS | Insights into cardiac metabolism pathways |
Pharmaceutical Synthesis | Clevidipine butyrate production monitoring | Enhanced synthesis efficiency using Raman spectroscopy |
Gut Health | Nutrient for colonocytes | Regulates immune response and oxidative stress |
Epigenetic Regulation | Histone deacetylase inhibition | Potential therapies for genetic/metabolic disorders |
Environmental Science | Bioremediation of chlorinated hydrocarbons | Effective treatment of contaminated aquifers |
Metabolic Disorders | Regulation of insulin sensitivity | Therapeutic potential for obesity and diabetes |
NASH Prevention | Modification of gut microbiome | Protective effects against metabolic disorders |
Mechanism of Action
The mechanism of action of chloromethyl butyrate involves its ability to act as an intermediate in various chemical reactions. It can form covalent bonds with other molecules, facilitating the synthesis of complex organic compounds. The molecular targets and pathways involved depend on the specific reactions and applications in which it is used.
Comparison with Similar Compounds
Structural and Physicochemical Properties
A comparative analysis of chloromethyl butyrate with structurally related esters reveals differences in molecular weight, boiling points, and functional group reactivity:
Compound | Molecular Formula | Molecular Weight | Boiling Point (°C) | Density (g/cm³) | Key Functional Groups |
---|---|---|---|---|---|
This compound | C₅H₉ClO₂ | 136.58 | Not reported | ~1.10 (est.) | Chloromethyl, butyrate ester |
β-Phenylethyl butyrate | C₁₂H₁₆O₂ | 192.25 | 270 | 1.002 | Phenyl, butyrate ester |
Chloromethyl isobutyrate | C₅H₉ClO₂ | 136.58 | Not reported | ~1.08 (est.) | Chloromethyl, isobutyrate |
Ethyl butyrate | C₆H₁₂O₂ | 116.16 | 121 | 0.879 | Ethyl, butyrate ester |
Methyl butyrate | C₅H₁₀O₂ | 102.13 | 102 | 0.898 | Methyl, butyrate ester |
Key Observations :
- Ethyl butyrate and methyl butyrate lack the chloromethyl group, making them less reactive in acyl transfer reactions but more volatile due to lower molecular weights .
- β-Phenylethyl butyrate incorporates a phenyl group, significantly increasing its molecular weight and boiling point, which suits it for fragrance applications .
Key Observations :
- This compound’s safety profile is less documented compared to ethyl butyrate and methyl butyrate , which have well-established workplace exposure limits .
- Chloromethyl chlorosulfate (a chlorinated sulfonic ester) exhibits severe corrosive properties and is regulated under strict handling protocols due to its lachrymatory effects .
Biological Activity
Chloromethyl butyrate (CMB) is a chemical compound with the formula CHClO. It is primarily recognized as an important intermediate in organic synthesis, particularly for producing substituted esters and other organic compounds. However, its biological activity, particularly in relation to its potential therapeutic effects and mechanisms of action, has garnered attention in recent research.
This compound is classified as an alkyl halide and is often utilized in synthetic organic chemistry due to its reactivity. The presence of the chloromethyl group allows it to participate in nucleophilic substitution reactions, making it a valuable building block for various synthetic pathways .
Potential Therapeutic Effects
Recent studies have suggested that compounds related to butyrate, including this compound, may exhibit various biological activities, particularly in the context of inflammation and cancer. Butyrate itself is known for its role as a short-chain fatty acid that influences gut health, modulates immune responses, and exhibits anti-inflammatory properties .
In Vitro Studies
In vitro studies have demonstrated that butyric acid can influence cellular processes such as apoptosis and differentiation in cancer cells. For instance, it has been shown to induce cell cycle arrest and promote apoptosis in various cancer cell lines . Although specific studies on this compound are scarce, the implications from related compounds suggest that CMB may also possess these properties.
Animal Models
Animal studies are essential for understanding the biological activity of this compound. Research involving butyrate administration has revealed significant reductions in colonic inflammation and tumorigenesis in models of inflammatory bowel disease and colorectal cancer . These findings highlight the potential for this compound to exert similar protective effects, warranting further investigation.
Data Table: Comparison of Biological Activities
Compound | Biological Activity | Mechanism of Action |
---|---|---|
This compound | Potential anti-inflammatory effects | Modulation of ROS levels; structural similarity to butyrate |
Butyric Acid | Anti-inflammatory; promotes apoptosis | Inhibition of NF-κB; histone deacetylase inhibition |
Other SCFAs | Modulate gut health; reduce inflammation | Influence on epithelial signaling pathways |
Q & A
Basic Research Questions
Q. What are the established methods for synthesizing chloromethyl butyrate, and how can its purity be confirmed?
this compound is typically synthesized via esterification reactions. For example, it has been used as a reagent in the synthesis of clevidipine butyrate by reacting with intermediates derived from diketene and 3-hydroxypropionitrile . To confirm purity, employ gas chromatography-mass spectrometry (GC-MS) with a polar capillary column (e.g., DB-5) and compare retention times to standards. Nuclear magnetic resonance (NMR) spectroscopy (¹H/¹³C) should confirm structural integrity, with characteristic peaks for the ester carbonyl (~170 ppm in ¹³C NMR) and chloromethyl group (~4.5 ppm in ¹H NMR) .
Q. Which analytical techniques are most effective for characterizing this compound?
Key techniques include:
- GC-MS : For assessing volatile impurities and quantifying purity using electron ionization (EI) at 70 eV.
- NMR Spectroscopy : ¹H NMR (400 MHz, CDCl₃) for integration of protons, and ¹³C NMR for carbonyl and chloromethyl group verification.
- Fourier-transform infrared spectroscopy (FTIR) : Peaks at ~1740 cm⁻¹ (C=O stretch) and ~750 cm⁻¹ (C-Cl stretch) .
- High-performance liquid chromatography (HPLC) : Use a C18 column with UV detection at 210 nm for non-volatile by-product analysis.
Q. What safety protocols are critical when handling this compound?
- Personal Protective Equipment (PPE) : Wear nitrile gloves, safety goggles, and a lab coat. Use a fume hood to minimize inhalation risks.
- Storage : Keep in airtight, light-resistant containers at 2–8°C, stabilized with potassium carbonate to prevent decomposition .
- Spill Management : Neutralize spills with sodium bicarbonate and absorb with inert materials (e.g., vermiculite) .
Advanced Research Questions
Q. How can reaction conditions be optimized for this compound in esterification processes?
Systematically vary:
- Catalysts : Compare p-toluenesulfonic acid (pTSA) vs. Lewis acids (e.g., ZnCl₂).
- Temperature : Test 0–25°C to minimize side reactions (e.g., hydrolysis).
- Molar Ratios : Optimize the alcohol-to-acid chloride ratio (e.g., 1.2:1) to drive esterification . Monitor progress via thin-layer chromatography (TLC) with hexane:ethyl acetate (4:1) as the mobile phase.
Q. How can by-products from this compound synthesis be identified and mitigated?
Common by-products include hydrolyzed derivatives (e.g., butyric acid) and dimerization products. Use LC-MS/MS in positive ion mode to detect non-volatile impurities. For volatile by-products, employ headspace GC-MS . Mitigation strategies:
- Drying Agents : Add molecular sieves (3Å) to absorb moisture.
- Low-Temperature Reactions : Conduct reactions below 10°C to suppress hydrolysis .
Q. What computational methods predict the reactivity and stability of this compound?
- Density Functional Theory (DFT) : Calculate bond dissociation energies (BDEs) for the C-Cl bond to assess hydrolytic stability.
- Molecular Dynamics (MD) Simulations : Model solvent interactions in polar aprotic solvents (e.g., dichloromethane).
- Retrosynthesis Tools : Use AI-driven platforms (e.g., Reaxys) to predict novel synthetic routes .
Q. How should researchers address discrepancies in reported physicochemical properties of this compound?
- Reproducibility Checks : Replicate synthesis and characterization methods from conflicting studies.
- Orthogonal Validation : Cross-verify melting points, boiling points, and spectral data using multiple techniques (e.g., differential scanning calorimetry for melting points) .
- Literature Review : Prioritize peer-reviewed journals over non-reviewed databases to resolve contradictions .
Q. How does this compound stability vary under different storage conditions, and how can this be systematically tested?
Design accelerated stability studies:
- Temperature : Store samples at 25°C, 4°C, and -20°C for 1–6 months.
- Light Exposure : Compare amber vs. clear glass containers under UV light (300–400 nm).
- Humidity : Test stability at 40–75% relative humidity using desiccators. Analyze degradation via GC-MS and ¹H NMR to quantify hydrolysis products. Stabilization with potassium carbonate has shown efficacy in reducing decomposition .
Properties
IUPAC Name |
chloromethyl butanoate | |
---|---|---|
Source | PubChem | |
URL | https://pubchem.ncbi.nlm.nih.gov | |
Description | Data deposited in or computed by PubChem | |
InChI |
InChI=1S/C5H9ClO2/c1-2-3-5(7)8-4-6/h2-4H2,1H3 | |
Source | PubChem | |
URL | https://pubchem.ncbi.nlm.nih.gov | |
Description | Data deposited in or computed by PubChem | |
InChI Key |
BDPZFQLKFUONAG-UHFFFAOYSA-N | |
Source | PubChem | |
URL | https://pubchem.ncbi.nlm.nih.gov | |
Description | Data deposited in or computed by PubChem | |
Canonical SMILES |
CCCC(=O)OCCl | |
Source | PubChem | |
URL | https://pubchem.ncbi.nlm.nih.gov | |
Description | Data deposited in or computed by PubChem | |
Molecular Formula |
C5H9ClO2 | |
Source | PubChem | |
URL | https://pubchem.ncbi.nlm.nih.gov | |
Description | Data deposited in or computed by PubChem | |
DSSTOX Substance ID |
DTXSID20369328 | |
Record name | Chloromethyl butyrate | |
Source | EPA DSSTox | |
URL | https://comptox.epa.gov/dashboard/DTXSID20369328 | |
Description | DSSTox provides a high quality public chemistry resource for supporting improved predictive toxicology. | |
Molecular Weight |
136.58 g/mol | |
Source | PubChem | |
URL | https://pubchem.ncbi.nlm.nih.gov | |
Description | Data deposited in or computed by PubChem | |
CAS No. |
33657-49-7 | |
Record name | Chloromethyl butyrate | |
Source | EPA DSSTox | |
URL | https://comptox.epa.gov/dashboard/DTXSID20369328 | |
Description | DSSTox provides a high quality public chemistry resource for supporting improved predictive toxicology. | |
Record name | chloromethyl butanoate | |
Source | European Chemicals Agency (ECHA) | |
URL | https://echa.europa.eu/information-on-chemicals | |
Description | The European Chemicals Agency (ECHA) is an agency of the European Union which is the driving force among regulatory authorities in implementing the EU's groundbreaking chemicals legislation for the benefit of human health and the environment as well as for innovation and competitiveness. | |
Explanation | Use of the information, documents and data from the ECHA website is subject to the terms and conditions of this Legal Notice, and subject to other binding limitations provided for under applicable law, the information, documents and data made available on the ECHA website may be reproduced, distributed and/or used, totally or in part, for non-commercial purposes provided that ECHA is acknowledged as the source: "Source: European Chemicals Agency, http://echa.europa.eu/". Such acknowledgement must be included in each copy of the material. ECHA permits and encourages organisations and individuals to create links to the ECHA website under the following cumulative conditions: Links can only be made to webpages that provide a link to the Legal Notice page. | |
Retrosynthesis Analysis
AI-Powered Synthesis Planning: Our tool employs the Template_relevance Pistachio, Template_relevance Bkms_metabolic, Template_relevance Pistachio_ringbreaker, Template_relevance Reaxys, Template_relevance Reaxys_biocatalysis model, leveraging a vast database of chemical reactions to predict feasible synthetic routes.
One-Step Synthesis Focus: Specifically designed for one-step synthesis, it provides concise and direct routes for your target compounds, streamlining the synthesis process.
Accurate Predictions: Utilizing the extensive PISTACHIO, BKMS_METABOLIC, PISTACHIO_RINGBREAKER, REAXYS, REAXYS_BIOCATALYSIS database, our tool offers high-accuracy predictions, reflecting the latest in chemical research and data.
Strategy Settings
Precursor scoring | Relevance Heuristic |
---|---|
Min. plausibility | 0.01 |
Model | Template_relevance |
Template Set | Pistachio/Bkms_metabolic/Pistachio_ringbreaker/Reaxys/Reaxys_biocatalysis |
Top-N result to add to graph | 6 |
Feasible Synthetic Routes
Disclaimer and Information on In-Vitro Research Products
Please be aware that all articles and product information presented on BenchChem are intended solely for informational purposes. The products available for purchase on BenchChem are specifically designed for in-vitro studies, which are conducted outside of living organisms. In-vitro studies, derived from the Latin term "in glass," involve experiments performed in controlled laboratory settings using cells or tissues. It is important to note that these products are not categorized as medicines or drugs, and they have not received approval from the FDA for the prevention, treatment, or cure of any medical condition, ailment, or disease. We must emphasize that any form of bodily introduction of these products into humans or animals is strictly prohibited by law. It is essential to adhere to these guidelines to ensure compliance with legal and ethical standards in research and experimentation.