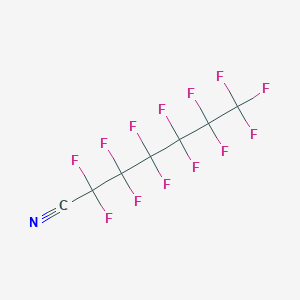
Perfluoroheptanenitrile
Overview
Description
Perfluoroheptanenitrile, with the chemical formula C7F13N , is a perfluorinated compound characterized by a fully fluorinated carbon chain and a nitrile group. This compound is known for its high thermal stability, chemical inertness, and unique physicochemical properties, making it valuable in various industrial and scientific applications .
Preparation Methods
Synthetic Routes and Reaction Conditions: Perfluoroheptanenitrile can be synthesized from tridecafluoroheptanoic acid amide. The process involves the dehydration of the amide using phosphorus pentoxide as a dehydrating agent. The reaction is carried out at a temperature of 250°C, and the product is obtained through direct distillation .
Industrial Production Methods: In industrial settings, the synthesis of this compound follows similar principles but on a larger scale. The reaction conditions are optimized to ensure high yield and purity. The use of advanced distillation techniques helps in the efficient separation and purification of the final product .
Chemical Reactions Analysis
Types of Reactions: Perfluoroheptanenitrile primarily undergoes substitution reactions due to the presence of the nitrile group. It can also participate in addition reactions under specific conditions.
Common Reagents and Conditions:
Substitution Reactions: These reactions often involve nucleophiles such as amines or alcohols. The reaction conditions typically include moderate temperatures and the presence of a catalyst to facilitate the substitution process.
Addition Reactions: These reactions may involve reagents like hydrogen or halogens under controlled conditions to add across the nitrile group.
Major Products Formed:
Substitution Reactions: The major products include substituted perfluoroheptanenitriles, where the nitrile group is replaced by other functional groups.
Addition Reactions: The products are typically perfluoroheptane derivatives with additional functional groups attached to the carbon chain.
Scientific Research Applications
Perfluoroheptanenitrile finds applications in various fields due to its unique properties:
Chemistry: It is used as a reagent in organic synthesis and as a precursor for the preparation of other fluorinated compounds.
Biology: Its inert nature makes it suitable for use in biological studies where non-reactive environments are required.
Medicine: Research is ongoing to explore its potential in drug delivery systems and as a component in medical devices.
Industry: It is utilized in the production of high-performance materials, including coatings, lubricants, and sealants, due to its chemical resistance and stability
Mechanism of Action
The mechanism by which perfluoroheptanenitrile exerts its effects is primarily through its chemical inertness and stability. The fully fluorinated carbon chain provides resistance to chemical reactions, making it an excellent candidate for applications requiring non-reactive environments. The nitrile group can participate in specific reactions, allowing for targeted modifications and functionalization .
Comparison with Similar Compounds
Perfluorohexyloctane: Another perfluorinated compound with similar properties but a different carbon chain length.
Perfluorooctanenitrile: Similar in structure but with a longer carbon chain.
Uniqueness: Perfluoroheptanenitrile stands out due to its specific carbon chain length and the presence of the nitrile group, which provides unique reactivity compared to other perfluorinated compounds. Its balance of chemical inertness and potential for functionalization makes it a versatile compound in various applications .
Properties
IUPAC Name |
2,2,3,3,4,4,5,5,6,6,7,7,7-tridecafluoroheptanenitrile | |
---|---|---|
Source | PubChem | |
URL | https://pubchem.ncbi.nlm.nih.gov | |
Description | Data deposited in or computed by PubChem | |
InChI |
InChI=1S/C7F13N/c8-2(9,1-21)3(10,11)4(12,13)5(14,15)6(16,17)7(18,19)20 | |
Source | PubChem | |
URL | https://pubchem.ncbi.nlm.nih.gov | |
Description | Data deposited in or computed by PubChem | |
InChI Key |
NBBJALWGNSZLRP-UHFFFAOYSA-N | |
Source | PubChem | |
URL | https://pubchem.ncbi.nlm.nih.gov | |
Description | Data deposited in or computed by PubChem | |
Canonical SMILES |
C(#N)C(C(C(C(C(C(F)(F)F)(F)F)(F)F)(F)F)(F)F)(F)F | |
Source | PubChem | |
URL | https://pubchem.ncbi.nlm.nih.gov | |
Description | Data deposited in or computed by PubChem | |
Molecular Formula |
C7F13N | |
Source | PubChem | |
URL | https://pubchem.ncbi.nlm.nih.gov | |
Description | Data deposited in or computed by PubChem | |
DSSTOX Substance ID |
DTXSID20382153 | |
Record name | Perfluoroheptanenitrile | |
Source | EPA DSSTox | |
URL | https://comptox.epa.gov/dashboard/DTXSID20382153 | |
Description | DSSTox provides a high quality public chemistry resource for supporting improved predictive toxicology. | |
Molecular Weight |
345.06 g/mol | |
Source | PubChem | |
URL | https://pubchem.ncbi.nlm.nih.gov | |
Description | Data deposited in or computed by PubChem | |
CAS No. |
62038-08-8 | |
Record name | Perfluoroheptanenitrile | |
Source | EPA DSSTox | |
URL | https://comptox.epa.gov/dashboard/DTXSID20382153 | |
Description | DSSTox provides a high quality public chemistry resource for supporting improved predictive toxicology. | |
Retrosynthesis Analysis
AI-Powered Synthesis Planning: Our tool employs the Template_relevance Pistachio, Template_relevance Bkms_metabolic, Template_relevance Pistachio_ringbreaker, Template_relevance Reaxys, Template_relevance Reaxys_biocatalysis model, leveraging a vast database of chemical reactions to predict feasible synthetic routes.
One-Step Synthesis Focus: Specifically designed for one-step synthesis, it provides concise and direct routes for your target compounds, streamlining the synthesis process.
Accurate Predictions: Utilizing the extensive PISTACHIO, BKMS_METABOLIC, PISTACHIO_RINGBREAKER, REAXYS, REAXYS_BIOCATALYSIS database, our tool offers high-accuracy predictions, reflecting the latest in chemical research and data.
Strategy Settings
Precursor scoring | Relevance Heuristic |
---|---|
Min. plausibility | 0.01 |
Model | Template_relevance |
Template Set | Pistachio/Bkms_metabolic/Pistachio_ringbreaker/Reaxys/Reaxys_biocatalysis |
Top-N result to add to graph | 6 |
Feasible Synthetic Routes
Disclaimer and Information on In-Vitro Research Products
Please be aware that all articles and product information presented on BenchChem are intended solely for informational purposes. The products available for purchase on BenchChem are specifically designed for in-vitro studies, which are conducted outside of living organisms. In-vitro studies, derived from the Latin term "in glass," involve experiments performed in controlled laboratory settings using cells or tissues. It is important to note that these products are not categorized as medicines or drugs, and they have not received approval from the FDA for the prevention, treatment, or cure of any medical condition, ailment, or disease. We must emphasize that any form of bodily introduction of these products into humans or animals is strictly prohibited by law. It is essential to adhere to these guidelines to ensure compliance with legal and ethical standards in research and experimentation.