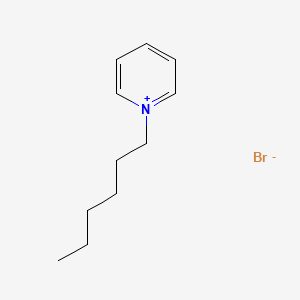
N-Hexylpyridinium bromide
Overview
Description
N-Hexylpyridinium bromide (CAS: 74440-81-6) is an ionic liquid and quaternary ammonium salt with the molecular formula C₁₁H₁₈BrN and a molecular weight of 244.17 g/mol . It is synthesized via N-alkylation of pyridine with 1-bromohexane under mild conditions, achieving near-complete quaternization as confirmed by ¹H-NMR spectroscopy . The compound features a hexyl chain attached to the pyridinium cation, balancing charge with a bromide anion.
Preparation Methods
Conventional Quaternization Synthesis
Reaction Overview
The most common preparation method for N-Hexylpyridinium bromide involves the quaternization of pyridine with hexyl bromide. This reaction typically proceeds by heating pyridine with hexyl bromide in an organic solvent under reflux conditions, facilitating nucleophilic substitution at the nitrogen atom of pyridine to form the quaternary ammonium salt.
Reaction Conditions
- Reactants: Pyridine and hexyl bromide in stoichiometric amounts.
- Solvents: Commonly used solvents include acetonitrile and ethanol.
- Temperature: Reflux temperature of the chosen solvent (e.g., ~82°C for ethanol, ~82°C for acetonitrile).
- Duration: Several hours (typically 4–8 hours) to ensure complete conversion.
- Post-reaction: The product is isolated by filtration or extraction and purified by recrystallization or distillation.
Industrial Scale Adaptations
In industrial production, the same synthetic route is scaled up using large reactors with controlled temperature and pressure to maximize yield and purity. Purification steps such as recrystallization or distillation are optimized for large volumes to remove residual reactants and by-products efficiently.
Parameter | Laboratory Scale | Industrial Scale |
---|---|---|
Solvent | Acetonitrile or ethanol | Same, with solvent recovery systems |
Temperature | Reflux (~82°C) | Controlled temperature and pressure |
Reaction Time | 4–8 hours | Optimized for throughput (shorter times) |
Purification Method | Recrystallization, distillation | Scaled recrystallization/distillation |
Yield | High (typically >90%) | High with process controls |
Solvent-Free and Greener Synthetic Methods
Solid-Phase Silica-Supported Alkylation
Recent research has explored solvent-free, greener methods for preparing substituted pyridinium bromides, which can be adapted for this compound synthesis. One such method involves the solid-phase alkylation of pyridine on silica support under muffle furnace heating. This approach offers several advantages:
- Reduced toxicity by avoiding organic solvents.
- Shorter reaction times (minutes rather than hours).
- Higher yields due to efficient energy transfer and reagent contact.
- Simplified product isolation.
Comparative Advantages
- Conventional method: Requires solvents, longer reaction times, and generates solvent waste.
- Solvent-free method: Environmentally friendly, faster, and potentially more cost-effective.
Example Procedure (Adapted)
- Mix pyridine and hexyl bromide with silica gel in appropriate molar ratios.
- Heat the mixture in a muffle furnace at controlled temperature (e.g., 100–150°C) for 30–60 minutes.
- Cool and extract the product using minimal solvent or direct isolation.
This method has been demonstrated with other pyridinium bromides and shows promise for this compound synthesis.
Anion Exchange and Purification Techniques
After synthesis, the bromide counterion in this compound can be exchanged with other anions (e.g., PF6^-, BF4^-, CF3SO3^-) to modify physicochemical properties. This is typically done by:
- Stirring the bromide salt with inorganic salts containing the desired anion in aqueous media.
- Room temperature reaction for 1–2 hours.
- Isolation of the new salt by filtration or crystallization.
This step is important for tailoring the compound for specific applications, such as ionic liquids or electrochemical additives.
Research Findings and Reaction Analysis
Reaction Mechanism
The quaternization proceeds via nucleophilic attack of the pyridine nitrogen lone pair on the electrophilic carbon of hexyl bromide, displacing bromide ion and forming the quaternary ammonium salt.
Reaction Yields and Kinetics
- Conventional reflux methods typically yield >90% pure product.
- Solvent-free methods can achieve comparable or higher yields in significantly shorter times.
- The presence of electron-withdrawing or donating groups on the pyridine ring or alkyl halide influences reaction rate and yield.
Summary Table of Preparation Methods
Method | Reactants | Solvent/Medium | Temperature & Time | Yield (%) | Advantages | Disadvantages |
---|---|---|---|---|---|---|
Conventional reflux | Pyridine + hexyl bromide | Acetonitrile/Ethanol | Reflux, 4–8 h | >90 | Well-established, high yield | Uses organic solvents, longer time |
Solvent-free silica-supported | Pyridine + hexyl bromide + silica | Solid phase, no solvent | 100–150°C, 30–60 min | 90–95 | Environmentally friendly, fast | Requires specialized equipment |
Industrial scale reflux | Pyridine + hexyl bromide | Acetonitrile/Ethanol | Controlled reflux, optimized | >90 | Scalable, controlled purity | Requires process control |
Anion exchange post-synthesis | This compound + inorganic salts | Aqueous solution | Room temp, 1–2 h | 94–98 | Tailors properties | Additional purification step |
Chemical Reactions Analysis
Types of Reactions: N-Hexylpyridinium bromide undergoes various chemical reactions, including:
Substitution Reactions: It can participate in nucleophilic substitution reactions where the bromide ion is replaced by other nucleophiles.
Oxidation and Reduction Reactions: It can undergo redox reactions, although these are less common compared to substitution reactions.
Common Reagents and Conditions:
Nucleophilic Substitution: Common reagents include sodium hydroxide, potassium cyanide, and other nucleophiles. The reactions are typically carried out in polar solvents such as water or alcohols.
Major Products:
Substitution Reactions: The major products depend on the nucleophile used. For example, using sodium hydroxide can yield N-hexylpyridinium hydroxide.
Oxidation Reactions: Oxidation can lead to the formation of pyridinium N-oxide derivatives.
Scientific Research Applications
Chemical Applications
N-Hexylpyridinium bromide serves as an effective solvent and catalyst in numerous organic reactions. Its ionic liquid properties facilitate reactions that may be challenging in conventional solvents. For instance, it has been utilized in substitution and oxidation reactions, leading to the formation of various derivatives such as N-hexylpyridinium hydroxide and pyridinium N-oxide derivatives.
Antimicrobial Properties
Research has highlighted the antimicrobial activity of this compound, particularly against Gram-positive and Gram-negative bacteria. The mechanism involves membrane disruption due to the interaction between the positively charged pyridinium moiety and negatively charged bacterial membranes, which increases permeability and leads to cell death .
Case Study: Surface Modification for Antimicrobial Activity
- Method : Poly(4-vinyl-N-hexylpyridinium bromide) was covalently attached to glass surfaces.
- Results : This modification resulted in surfaces that could kill over 94% of Staphylococcus aureus upon contact, showcasing its potential for use in antimicrobial coatings for medical devices .
Drug Delivery Systems
This compound has been explored for its potential as a drug delivery agent. Its ability to interact with biological membranes makes it a candidate for enhancing drug permeability across cellular barriers.
Industrial Applications
In industrial settings, this compound is employed as a corrosion inhibitor for metals, especially in acidic environments. This property is crucial for protecting metal surfaces from degradation due to corrosive agents.
Separation Science
Recent studies suggest that this compound can be utilized in separating azeotropes—mixtures of liquids that exhibit a constant boiling point. Its thermodynamic properties make it suitable for applications in separation science, enhancing efficiency in chemical processes .
Comprehensive Data Tables
Bacteria | % Reduction in Viable Cells |
---|---|
Staphylococcus aureus | >94% |
Escherichia coli | >99% |
Pseudomonas aeruginosa | >99% |
Staphylococcus epidermidis | >99% |
Case Study: Biocompatibility Concerns
While this compound demonstrates strong antimicrobial properties, studies have raised concerns about its biocompatibility. A composite material incorporating this compound showed significant activation of blood platelets, suggesting caution for applications involving direct contact with blood .
Future Research Directions
Ongoing research is focusing on improving the biocompatibility of materials containing this compound while maintaining their antimicrobial efficacy. This includes exploring copolymers that may enhance compatibility with mammalian cells without compromising their antimicrobial properties .
Mechanism of Action
The mechanism of action of N-Hexylpyridinium bromide involves its interaction with biological membranes and metal surfaces:
Comparison with Similar Compounds
Comparison with Similar Alkylpyridinium Bromides
Alkylpyridinium bromides differ primarily in alkyl chain length, which critically influences physicochemical properties, biological activity, and applications. Below is a comparative analysis of N-hexylpyridinium bromide (C6) with shorter (C4) and longer-chain analogs (C12, C14, C16):
Table 1: Structural and Spectral Comparison of Alkylpyridinium Bromides
Key Findings:
Alkyl Chain Impact on Solubility and Toxicity :
- Shorter chains (C4–C6) enhance water solubility due to reduced hydrophobicity, whereas longer chains (C12–C16) exhibit stronger surfactant behavior but higher aquatic toxicity .
- This compound (C6) strikes a balance between solubility and membrane permeability, making it suitable for biomedical applications .
Spectral Trends :
- Pyridinium ring protons (δ 9.14–8.11 ppm) remain consistent across analogs, but alkyl chain protons (δ 1.23–0.83 ppm) show increased multiplicity and integration with chain length .
Biological Activity :
- Longer chains (e.g., C16) demonstrate superior antimicrobial efficacy but higher cytotoxicity, limiting their use in vivo. This compound (C6) provides moderate antimicrobial action with lower ecological risk .
Comparison with Other Bromide Salts
Sepantronium Bromide (YM-155)
While both compounds are bromide salts, Sepantronium bromide (C₂₀H₁₉BrN₄O₃) is structurally distinct, featuring a complex heterocyclic scaffold. Key differences include:
- Application: Sepantronium is a survivin inhibitor with potent anticancer activity (IC₅₀ = 0.54 nM in PC-3 cells) , whereas this compound is non-pharmacological and used in materials science.
- Toxicity : Sepantronium shows targeted tumor distribution but risks neurotoxicity at high doses , while this compound’s toxicity is primarily environmental .
Counterion Variants (e.g., Cl⁻, BF₄⁻)
N-Hexylpyridinium salts with alternative anions (e.g., chloride, tetrafluoroborate) exhibit distinct properties:
Biological Activity
N-Hexylpyridinium bromide (NHPB) is a quaternary ammonium compound with significant biological activity, particularly noted for its antimicrobial properties. This article explores the compound's biological mechanisms, applications, and relevant research findings.
- Molecular Formula : C₁₁H₁₈BrN
- IUPAC Name : this compound
- CAS Number : 74440-81-6
NHPB is an ionic liquid characterized by high thermal stability, low volatility, and excellent solubility in both water and organic solvents. Its structure allows it to function effectively as a surfactant, stabilizing colloidal systems and influencing biological interactions.
NHPB exhibits its antimicrobial activity primarily through the disruption of bacterial cell membranes. The positively charged pyridinium moiety interacts with negatively charged components of bacterial membranes, leading to increased permeability and eventual cell lysis. This mechanism is crucial for its effectiveness against a range of microorganisms, including Gram-positive and Gram-negative bacteria.
Antimicrobial Activity
Numerous studies have documented the antimicrobial efficacy of NHPB. Below is a summary of key findings:
Case Studies and Research Findings
- Antimicrobial Surfaces : Research by Allison et al. demonstrated that copolymer films incorporating NHPB exhibited antimicrobial properties while also being biocompatible at lower concentrations. However, higher concentrations led to significant activation and disruption of blood platelets, raising concerns about their use in medical applications involving direct blood contact .
- Polymer Composites : A study explored the use of NHPB in creating antimicrobial surfaces that effectively killed airborne bacteria on contact. The surfaces modified with NHPB showed a remarkable ability to reduce viable bacterial cells significantly compared to untreated materials .
- Biocompatibility Concerns : While NHPB has strong antimicrobial properties, its biocompatibility varies with concentration. At lower levels, it poses minimal risk; however, higher concentrations can lead to adverse effects on mammalian cells . Research indicates that polymers containing high percentages of NHPB may not be suitable for applications involving direct contact with blood or tissues .
Applications
NHPB's unique properties make it suitable for various applications:
- Antimicrobial Coatings : Used in coatings for medical devices and surfaces to prevent bacterial colonization.
- Drug Delivery Systems : Investigated for its potential to enhance drug delivery through biological membranes due to its surfactant properties.
- Corrosion Inhibitors : Applied in industrial settings to protect metals from corrosion in acidic environments.
Q & A
Basic Research Questions
Q. What are the standard protocols for synthesizing and purifying N-Hexylpyridinium bromide, and how can purity be validated?
this compound is synthesized via quaternization of pyridine with 1-bromohexane under reflux (70°C, 72 hours) in a solvent-free system. Post-synthesis, unreacted reagents are removed by repeated ethyl acetate washes, followed by vacuum drying. Purity validation involves nuclear magnetic resonance (NMR) for structural confirmation, Fourier-transform infrared spectroscopy (FTIR) for functional group analysis, and elemental analysis (C, H, N, Br) to verify stoichiometry .
Q. What physicochemical properties are critical for characterizing this compound in research applications?
Key properties include thermal stability (analyzed via thermogravimetric analysis (TGA) and differential scanning calorimetry (DSC)), solubility in polar/non-polar solvents, and hygroscopicity. Molecular weight (244.17 g/mol) and ionic conductivity are also relevant for applications in catalysis or electrochemistry. Techniques like Karl Fischer titration quantify water content, which can affect reactivity .
Advanced Research Questions
Q. How does this compound influence reaction mechanisms in catalytic systems, and what methodologies can elucidate its role?
As a solvent and catalyst, it stabilizes transition states via electrostatic interactions and hydrogen bonding. Mechanistic studies require kinetic profiling (e.g., varying substrate/catalyst ratios) coupled with computational modeling (density functional theory (DFT)) to map energy barriers. Spectroscopic methods like Raman or UV-Vis can track intermediate formation. Comparative studies with shorter-chain analogs (e.g., N-butylpyridinium bromide) isolate chain-length effects .
Q. What experimental approaches are recommended to resolve contradictions in reported antimicrobial efficacy data for this compound?
Discrepancies may arise from variations in bacterial strains, concentration thresholds, or assay conditions (e.g., broth microdilution vs. agar diffusion). Standardized protocols (CLSI guidelines) and minimum inhibitory concentration (MIC) assays under controlled pH/temperature are critical. Scanning electron microscopy (SEM) can visualize membrane disruption, while fluorescence assays (e.g., propidium iodide uptake) confirm cell permeability changes .
Q. How can researchers optimize this compound's biocompatibility without compromising its antimicrobial activity?
Hybridization with biocompatible polymers (e.g., polyethylene glycol) or encapsulation in silica nanoparticles reduces cytotoxicity. Hemolysis assays (using red blood cells) and MTT assays (on mammalian cell lines) quantify biocompatibility. Structure-activity relationship (SAR) studies comparing alkyl chain lengths and counterion effects (e.g., bromide vs. tetrafluoroborate) identify toxicity drivers .
Q. What computational strategies are effective for modeling this compound's interactions in enzymatic systems?
Molecular dynamics (MD) simulations with force fields (e.g., AMBER or CHARMM) model cation-anion interactions around enzymes. Radial distribution function (RDF) analysis identifies proximity between bromide ions and enzyme surfaces, while root-mean-square deviation (RMSD) tracks structural stability. Free energy calculations (e.g., MMPBSA) quantify binding affinities .
Q. How should researchers address inconsistencies in bromide ion quantification across analytical methods?
Cross-validate results using ion chromatography (IC), automated fluorescein assays, and inductively coupled plasma mass spectrometry (ICP-MS). Account for matrix effects (e.g., organic solvents) and calibrate with certified reference materials. Statistical analysis (paired t-tests) ensures method equivalence, as demonstrated in bromide recovery studies .
Q. What advanced techniques characterize solvent-substrate interactions in ionic liquid-mediated reactions?
Neutron scattering or X-ray diffraction reveals bulk solvent structure. Nuclear Overhauser effect spectroscopy (NOESY) NMR detects spatial proximity between ionic liquid cations and substrates. Transient absorption spectroscopy monitors electron transfer dynamics in photochemical reactions .
Notes
- Avoid commercial databases (e.g., BenchChem) for physicochemical data; prioritize peer-reviewed studies or institutional reports.
- For toxicity assessments, adhere to OECD guidelines for in vitro and in vivo testing.
- Computational models should validate force fields against experimental data (e.g., RDF profiles from MD vs. X-ray crystallography).
Properties
IUPAC Name |
1-hexylpyridin-1-ium;bromide | |
---|---|---|
Source | PubChem | |
URL | https://pubchem.ncbi.nlm.nih.gov | |
Description | Data deposited in or computed by PubChem | |
InChI |
InChI=1S/C11H18N.BrH/c1-2-3-4-6-9-12-10-7-5-8-11-12;/h5,7-8,10-11H,2-4,6,9H2,1H3;1H/q+1;/p-1 | |
Source | PubChem | |
URL | https://pubchem.ncbi.nlm.nih.gov | |
Description | Data deposited in or computed by PubChem | |
InChI Key |
SZRSEFNUSHACPD-UHFFFAOYSA-M | |
Source | PubChem | |
URL | https://pubchem.ncbi.nlm.nih.gov | |
Description | Data deposited in or computed by PubChem | |
Canonical SMILES |
CCCCCC[N+]1=CC=CC=C1.[Br-] | |
Source | PubChem | |
URL | https://pubchem.ncbi.nlm.nih.gov | |
Description | Data deposited in or computed by PubChem | |
Molecular Formula |
C11H18BrN | |
Source | PubChem | |
URL | https://pubchem.ncbi.nlm.nih.gov | |
Description | Data deposited in or computed by PubChem | |
DSSTOX Substance ID |
DTXSID7047934 | |
Record name | 1-Hexylpyridinium bromide | |
Source | EPA DSSTox | |
URL | https://comptox.epa.gov/dashboard/DTXSID7047934 | |
Description | DSSTox provides a high quality public chemistry resource for supporting improved predictive toxicology. | |
Molecular Weight |
244.17 g/mol | |
Source | PubChem | |
URL | https://pubchem.ncbi.nlm.nih.gov | |
Description | Data deposited in or computed by PubChem | |
CAS No. |
74440-81-6 | |
Record name | 1-Hexylpyridinium bromide | |
Source | EPA DSSTox | |
URL | https://comptox.epa.gov/dashboard/DTXSID7047934 | |
Description | DSSTox provides a high quality public chemistry resource for supporting improved predictive toxicology. | |
Record name | 1-Hexylpyridinium bromide | |
Source | FDA Global Substance Registration System (GSRS) | |
URL | https://gsrs.ncats.nih.gov/ginas/app/beta/substances/N7AT59Q225 | |
Description | The FDA Global Substance Registration System (GSRS) enables the efficient and accurate exchange of information on what substances are in regulated products. Instead of relying on names, which vary across regulatory domains, countries, and regions, the GSRS knowledge base makes it possible for substances to be defined by standardized, scientific descriptions. | |
Explanation | Unless otherwise noted, the contents of the FDA website (www.fda.gov), both text and graphics, are not copyrighted. They are in the public domain and may be republished, reprinted and otherwise used freely by anyone without the need to obtain permission from FDA. Credit to the U.S. Food and Drug Administration as the source is appreciated but not required. | |
Synthesis routes and methods
Procedure details
Retrosynthesis Analysis
AI-Powered Synthesis Planning: Our tool employs the Template_relevance Pistachio, Template_relevance Bkms_metabolic, Template_relevance Pistachio_ringbreaker, Template_relevance Reaxys, Template_relevance Reaxys_biocatalysis model, leveraging a vast database of chemical reactions to predict feasible synthetic routes.
One-Step Synthesis Focus: Specifically designed for one-step synthesis, it provides concise and direct routes for your target compounds, streamlining the synthesis process.
Accurate Predictions: Utilizing the extensive PISTACHIO, BKMS_METABOLIC, PISTACHIO_RINGBREAKER, REAXYS, REAXYS_BIOCATALYSIS database, our tool offers high-accuracy predictions, reflecting the latest in chemical research and data.
Strategy Settings
Precursor scoring | Relevance Heuristic |
---|---|
Min. plausibility | 0.01 |
Model | Template_relevance |
Template Set | Pistachio/Bkms_metabolic/Pistachio_ringbreaker/Reaxys/Reaxys_biocatalysis |
Top-N result to add to graph | 6 |
Feasible Synthetic Routes
Disclaimer and Information on In-Vitro Research Products
Please be aware that all articles and product information presented on BenchChem are intended solely for informational purposes. The products available for purchase on BenchChem are specifically designed for in-vitro studies, which are conducted outside of living organisms. In-vitro studies, derived from the Latin term "in glass," involve experiments performed in controlled laboratory settings using cells or tissues. It is important to note that these products are not categorized as medicines or drugs, and they have not received approval from the FDA for the prevention, treatment, or cure of any medical condition, ailment, or disease. We must emphasize that any form of bodily introduction of these products into humans or animals is strictly prohibited by law. It is essential to adhere to these guidelines to ensure compliance with legal and ethical standards in research and experimentation.