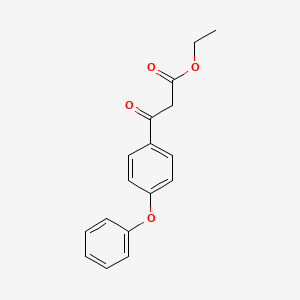
Ethyl 3-oxo-3-(4-phenoxyphenyl)propanoate
Overview
Description
Ethyl 3-oxo-3-(4-phenoxyphenyl)propanoate is a chemical compound with the molecular formula C17H16O4 . It has an average mass of 284.306 Da and a monoisotopic mass of 284.104858 Da .
Molecular Structure Analysis
The molecular structure of Ethyl 3-oxo-3-(4-phenoxyphenyl)propanoate consists of a propanoate backbone with a 3-oxo group and a 4-phenoxyphenyl group . The 3-oxo group indicates the presence of a carbonyl group (C=O) on the third carbon of the propanoate backbone, while the 4-phenoxyphenyl group is a phenyl ring attached to the fourth carbon of the propanoate backbone via an oxygen atom .Scientific Research Applications
Green Chemistry Applications
- Polymer Synthesis : A study explored using phloretic acid, a phenolic compound, as a renewable building block for enhancing the reactivity of molecules towards benzoxazine ring formation, offering a sustainable alternative to phenol. This approach indicates the potential for ethyl 3-oxo-3-(4-phenoxyphenyl)propanoate in synthesizing bio-based polymers with applications in materials science (Acerina Trejo-Machin et al., 2017).
Catalysis and Reaction Media
- Catalytic Activity : A study on poly(3,4-ethylenedioxythiophene) demonstrated its use in immobilizing metal particle catalysts and reagents for catalytic activities in hydrogenation and electro-oxidation reactions. This suggests potential uses for ethyl 3-oxo-3-(4-phenoxyphenyl)propanoate in developing new catalytic systems or reaction media (C. Sivakumar & K. Phani, 2011).
Environmental Bioremediation
- Bioremediation : Research on Fusarium incarnatum UC-14's laccase in a reverse micelles system for bisphenol A degradation highlights the enzyme's effectiveness in degrading hydrophobic phenolic pollutants. This points towards the potential role of similar compounds in enhancing biodegradability of environmental pollutants (Urvish Chhaya & A. Gupte, 2013).
Drug Synthesis Intermediates
- Pharmaceutical Intermediates : A study on the enzyme-catalyzed synthesis of (R)- and (S)-3-hydroxy-3-(10-alkyl-10H-phenothiazin-3-yl)propanoic acids from racemic ethyl 3-hydroxy-3-(10-alkyl-10H-phenothiazin-3-yl)propanoates reveals the efficiency of multienzymatic procedures in producing enantiomerically enriched acids, which are valuable in drug synthesis (J. Brem et al., 2010).
Analytical Chemistry
- Analytical Characterization : Studies on polymorphism in pharmaceutical compounds, including a detailed analysis of ethyl 3-{3-[((2R)-3-{[2-(2,3-dihydro-1H-inden-2-yl)-1,1-dimethylethyl]amino}-2-hydroxypropyl)oxy]-4,5-difluorophenyl}propanoate hydrochloride, reveal the complexity in characterizing such molecules using spectroscopic and diffractometric techniques. This underscores the relevance of ethyl 3-oxo-3-(4-phenoxyphenyl)propanoate in pharmaceutical analysis and development (F. Vogt et al., 2013).
properties
IUPAC Name |
ethyl 3-oxo-3-(4-phenoxyphenyl)propanoate | |
---|---|---|
Source | PubChem | |
URL | https://pubchem.ncbi.nlm.nih.gov | |
Description | Data deposited in or computed by PubChem | |
InChI |
InChI=1S/C17H16O4/c1-2-20-17(19)12-16(18)13-8-10-15(11-9-13)21-14-6-4-3-5-7-14/h3-11H,2,12H2,1H3 | |
Source | PubChem | |
URL | https://pubchem.ncbi.nlm.nih.gov | |
Description | Data deposited in or computed by PubChem | |
InChI Key |
CAXNCSRMNJVMKD-UHFFFAOYSA-N | |
Source | PubChem | |
URL | https://pubchem.ncbi.nlm.nih.gov | |
Description | Data deposited in or computed by PubChem | |
Canonical SMILES |
CCOC(=O)CC(=O)C1=CC=C(C=C1)OC2=CC=CC=C2 | |
Source | PubChem | |
URL | https://pubchem.ncbi.nlm.nih.gov | |
Description | Data deposited in or computed by PubChem | |
Molecular Formula |
C17H16O4 | |
Source | PubChem | |
URL | https://pubchem.ncbi.nlm.nih.gov | |
Description | Data deposited in or computed by PubChem | |
DSSTOX Substance ID |
DTXSID10375079 | |
Record name | ethyl 3-oxo-3-(4-phenoxyphenyl)propanoate | |
Source | EPA DSSTox | |
URL | https://comptox.epa.gov/dashboard/DTXSID10375079 | |
Description | DSSTox provides a high quality public chemistry resource for supporting improved predictive toxicology. | |
Molecular Weight |
284.31 g/mol | |
Source | PubChem | |
URL | https://pubchem.ncbi.nlm.nih.gov | |
Description | Data deposited in or computed by PubChem | |
Product Name |
Ethyl 3-oxo-3-(4-phenoxyphenyl)propanoate | |
CAS RN |
59447-12-0 | |
Record name | ethyl 3-oxo-3-(4-phenoxyphenyl)propanoate | |
Source | EPA DSSTox | |
URL | https://comptox.epa.gov/dashboard/DTXSID10375079 | |
Description | DSSTox provides a high quality public chemistry resource for supporting improved predictive toxicology. | |
Synthesis routes and methods I
Procedure details
Synthesis routes and methods II
Procedure details
Retrosynthesis Analysis
AI-Powered Synthesis Planning: Our tool employs the Template_relevance Pistachio, Template_relevance Bkms_metabolic, Template_relevance Pistachio_ringbreaker, Template_relevance Reaxys, Template_relevance Reaxys_biocatalysis model, leveraging a vast database of chemical reactions to predict feasible synthetic routes.
One-Step Synthesis Focus: Specifically designed for one-step synthesis, it provides concise and direct routes for your target compounds, streamlining the synthesis process.
Accurate Predictions: Utilizing the extensive PISTACHIO, BKMS_METABOLIC, PISTACHIO_RINGBREAKER, REAXYS, REAXYS_BIOCATALYSIS database, our tool offers high-accuracy predictions, reflecting the latest in chemical research and data.
Strategy Settings
Precursor scoring | Relevance Heuristic |
---|---|
Min. plausibility | 0.01 |
Model | Template_relevance |
Template Set | Pistachio/Bkms_metabolic/Pistachio_ringbreaker/Reaxys/Reaxys_biocatalysis |
Top-N result to add to graph | 6 |
Feasible Synthetic Routes
Disclaimer and Information on In-Vitro Research Products
Please be aware that all articles and product information presented on BenchChem are intended solely for informational purposes. The products available for purchase on BenchChem are specifically designed for in-vitro studies, which are conducted outside of living organisms. In-vitro studies, derived from the Latin term "in glass," involve experiments performed in controlled laboratory settings using cells or tissues. It is important to note that these products are not categorized as medicines or drugs, and they have not received approval from the FDA for the prevention, treatment, or cure of any medical condition, ailment, or disease. We must emphasize that any form of bodily introduction of these products into humans or animals is strictly prohibited by law. It is essential to adhere to these guidelines to ensure compliance with legal and ethical standards in research and experimentation.