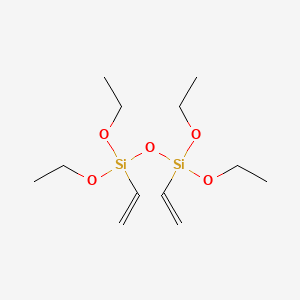
1,3-Divinyltetraethoxydisiloxane
Overview
Description
1,3-Divinyltetraethoxydisiloxane is an organosilicon compound with the molecular formula C10H22O4Si2. It is a colorless liquid that is used in various chemical reactions and industrial applications. The compound is characterized by the presence of vinyl groups attached to silicon atoms, which makes it a versatile reagent in organic synthesis.
Preparation Methods
Synthetic Routes and Reaction Conditions
1,3-Divinyltetraethoxydisiloxane can be synthesized through the hydrosilylation reaction of vinyl-containing silanes with tetraethoxysilane. The reaction typically involves the use of a platinum catalyst, such as Karstedt’s catalyst, under controlled temperature and pressure conditions. The reaction proceeds as follows: [ \text{CH}_2=\text{CH}-\text{Si}(\text{OEt})_3 + \text{H}-\text{Si}(\text{OEt})_3 \xrightarrow{\text{Pt catalyst}} \text{CH}_2=\text{CH}-\text{Si}(\text{OEt})_2-\text{Si}(\text{OEt})_2-\text{CH}=\text{CH}_2 ]
Industrial Production Methods
Industrial production of this compound involves large-scale hydrosilylation processes using continuous flow reactors. The use of high-purity starting materials and efficient catalysts ensures high yields and purity of the final product. The reaction conditions are optimized to minimize side reactions and maximize the efficiency of the process.
Chemical Reactions Analysis
Types of Reactions
1,3-Divinyltetraethoxydisiloxane undergoes various types of chemical reactions, including:
Hydrosilylation: Addition of silicon-hydrogen bonds to carbon-carbon double bonds.
Cross-coupling reactions: Formation of carbon-carbon bonds using palladium or nickel catalysts.
Polymerization: Formation of polymers through the reaction of vinyl groups with other monomers.
Common Reagents and Conditions
Hydrosilylation: Platinum catalysts, such as Karstedt’s catalyst, are commonly used. The reaction is typically carried out at room temperature or slightly elevated temperatures.
Cross-coupling reactions: Palladium or nickel catalysts, along with ligands such as triphenylphosphine, are used. The reaction conditions vary depending on the specific coupling partners.
Polymerization: Radical initiators or transition metal catalysts are used to initiate the polymerization process.
Major Products Formed
Hydrosilylation: The major product is the addition product where the silicon-hydrogen bond has added across the carbon-carbon double bond.
Cross-coupling reactions: The major products are the coupled products where new carbon-carbon bonds have been formed.
Polymerization: The major products are polymers with varying molecular weights and properties, depending on the monomers used.
Scientific Research Applications
Scientific Research Applications
-
Polymer Chemistry
- DVTES is primarily employed in the synthesis of silicone-based polymers. Its ability to act as a vinyl donor facilitates the formation of cross-linked structures, which are crucial for developing elastomers and sealants. The compound is often used in creating polydimethylsiloxanes (PDMS), which have applications in coatings, adhesives, and biomedical devices.
-
Catalysis
- DVTES is utilized as a ligand in organometallic chemistry, particularly in the formation of platinum-based catalysts such as Karstedt's catalyst. These catalysts are vital for hydrosilylation reactions, which are essential in producing silicone materials with specific properties . The catalytic efficiency of these complexes has been demonstrated in various studies, showing high turnover numbers (TON) in synthetic reactions .
-
Biomedical Applications
- Research has explored DVTES for its potential to enhance biocompatibility in medical devices. By modifying surfaces with DVTES-derived polymers, researchers aim to reduce protein adsorption and improve the performance of implants . Studies have shown that grafting hydrophilic polymers onto surfaces treated with DVTES can yield biocompatible coatings that minimize thrombogenicity .
- Electrochemical Applications
Table 1: Comparison of Applications of DVTES
Application Area | Description | Key Benefits |
---|---|---|
Polymer Chemistry | Synthesis of silicone-based polymers | Cross-linking capabilities for enhanced durability |
Catalysis | Formation of platinum-based catalysts | High catalytic efficiency in hydrosilylation |
Biomedical Devices | Surface modification for implants | Improved biocompatibility and reduced thrombogenicity |
Electrochemistry | Enhancing properties of lithium-ion battery materials | Increased conductivity and performance |
Case Studies
- Hydrosilylation Reactions : A study demonstrated that platinum-DVTES complexes exhibited exceptional catalytic activity in hydrosilylation reactions, achieving yields above 95% with minimal by-products. This efficiency highlights the importance of DVTES in industrial applications where high purity and selectivity are required .
- Biocompatible Coatings : Research involving the grafting of DVTES-derived polymers onto metal surfaces showed a significant reduction in fibrinogen adsorption compared to untreated surfaces. This finding supports the use of DVTES in developing medical devices that require biocompatible interfaces .
- Battery Performance : Investigations into lithium-rich layered oxide cathodes revealed that incorporating DVTES into the electrode material resulted in improved electrochemical stability and capacity retention during cycling tests. This application is particularly relevant for next-generation battery technologies aimed at enhancing energy density .
Mechanism of Action
The mechanism of action of 1,3-divinyltetraethoxydisiloxane in hydrosilylation reactions involves the activation of the silicon-hydrogen bond by the platinum catalyst. The activated silicon-hydrogen bond then adds across the carbon-carbon double bond, forming the addition product. In cross-coupling reactions, the compound acts as a vinyl donor, participating in the formation of new carbon-carbon bonds through the catalytic cycle of the palladium or nickel catalyst.
Comparison with Similar Compounds
1,3-Divinyltetraethoxydisiloxane can be compared with other similar compounds, such as:
1,1,3,3-Tetramethyl-1,3-divinyldisiloxane: This compound has methyl groups instead of ethoxy groups attached to the silicon atoms. It is also used in hydrosilylation and cross-coupling reactions but has different reactivity and properties due to the presence of methyl groups.
1,3-Divinyltetramethyldisiloxane: Similar to 1,1,3,3-tetramethyl-1,3-divinyldisiloxane, this compound has methyl groups instead of ethoxy groups. It is used in similar applications but has different solubility and reactivity properties.
The uniqueness of this compound lies in its ethoxy groups, which provide different solubility and reactivity characteristics compared to its methyl-substituted counterparts. This makes it a valuable reagent in specific applications where these properties are advantageous.
Biological Activity
1,3-Divinyltetraethoxydisiloxane (DVTES) is a siloxane compound characterized by its unique molecular structure, which includes two vinyl groups attached to a tetrasiloxane backbone. This compound exhibits significant potential in various industrial applications, particularly in polymer synthesis and material science. However, its biological activity remains less explored. This article aims to provide a comprehensive overview of the biological activity of DVTES, including its chemical properties, synthesis methods, and relevant case studies.
- Molecular Formula : C₈H₁₈O₂Si₂
- Molecular Weight : 186.4 g/mol
- Density : 0.809 g/mL at 25 °C
- Boiling Point : 139-140 °C
- Melting Point : -99 °C
- Flash Point : 24 °C (75 °F)
DVTES is a colorless or yellowish transparent liquid that is moisture-sensitive and insoluble in water, which necessitates careful handling due to its flammability and irritant properties .
Synthesis Methods
DVTES can be synthesized through several methods, including:
- Hydrosilylation Reactions : Involving the addition of vinyl-containing silanes to siloxanes.
- Cross-Coupling Reactions : Utilizing DVTES as a vinyl donor in reactions with various catalysts to form more complex siloxane structures.
These methods allow for the production of high-purity DVTES suitable for both research and industrial applications .
Biological Activity
While the primary focus of research on DVTES has been on its chemical reactivity and applications in material science, some studies have explored its biological interactions:
Case Studies and Research Findings
- Toxicological Assessment :
-
Polymer Biocatalysis :
- Research highlighted the use of DVTES in modifying polysiloxane resins, which could have implications for biocompatibility in medical applications. The study noted that the incorporation of DVTES improved the mechanical properties of the resulting materials, suggesting potential use in biomedical devices .
- Electrochemical Applications :
Comparative Analysis with Similar Compounds
To better understand the unique properties of DVTES, a comparison with structurally similar compounds is useful:
Compound Name | Molecular Formula | Key Characteristics |
---|---|---|
1,3-Divinyl-1,1,3,3-tetramethylsiloxane | C₈H₁₈OSi₂ | Similar structure; used in polymerization |
Vinyltrimethoxysilane | C₈H₁₈O₃Si | More reactive due to methoxy groups; used as a coupling agent |
Divinylbenzene | C₈H₈ | Aromatic compound; primarily for polymerization reactions |
Octamethylcyclotetrasiloxane | C₈H₂₄OSi₄ | Less reactive than divinyl compounds; used in silicone products |
DVTES's unique dual vinyl groups enable specific reactivity patterns advantageous in silicone chemistry and advanced material synthesis compared to these similar compounds .
Q & A
Basic Research Questions
Q. What are the critical safety considerations when handling 1,3-Divinyltetraethoxydisiloxane in laboratory settings?
- Methodological Answer : Due to its classification as a flammable liquid (flash point: 24°C), handling must occur in well-ventilated areas away from ignition sources. Use non-sparking tools and ground equipment to prevent static discharge. Personal protective equipment (PPE), including nitrile gloves, chemical-resistant goggles, and flame-retardant lab coats, is mandatory. Spills should be contained using inert absorbents (e.g., sand) and disposed of via approved waste management protocols .
Q. How should this compound be stored to maintain stability?
- Methodological Answer : Store in tightly sealed containers under inert gas (e.g., nitrogen) to prevent moisture absorption and oxidation. Maintain temperatures below 25°C in a fireproof cabinet separate from oxidizers. Regularly inspect containers for leaks, and ensure storage areas are equipped with explosion-proof ventilation .
Q. What first-aid measures are recommended for accidental exposure?
- Methodological Answer :
- Inhalation : Move to fresh air; administer oxygen if breathing is labored.
- Skin contact : Wash immediately with soap and water; remove contaminated clothing.
- Eye exposure : Rinse with water for 15 minutes; seek ophthalmological evaluation.
- Ingestion : Do not induce vomiting; provide medical attention for possible aspiration hazards .
Q. What analytical techniques are suitable for characterizing this compound?
- Methodological Answer : Use gas chromatography-mass spectrometry (GC-MS) for purity assessment (CAS 2627-95-4). Infrared (IR) spectroscopy can confirm functional groups (e.g., Si-O-Si and vinyl stretches). Nuclear magnetic resonance (NMR) (¹H, ¹³C, ²⁹Si) resolves structural details, while elemental analysis verifies molecular composition (C8H18O4Si2) .
Advanced Research Questions
Q. How can Grubbs’ catalyst be optimized for copolymerizing this compound with dienes?
- Methodological Answer : In ADMET copolymerization, use a catalyst loading of 0.5–1.0 mol% under inert conditions (argon). React at 40–60°C in anhydrous toluene to balance reaction rate and thermal stability. Monitor cross-linking via gel permeation chromatography (GPC) and adjust monomer feed ratios to control polymer branching .
Q. What strategies mitigate discrepancies in hydrosilylation reaction yields involving this compound?
- Methodological Answer : Yield variations may arise from trace moisture or oxygen. Pre-dry substrates with molecular sieves and employ Schlenk-line techniques. Use platinum-based catalysts (e.g., Karstedt’s catalyst) at 0.01–0.1 wt% to enhance selectivity. Kinetic studies via in-situ FTIR can identify side reactions (e.g., homopolymerization) .
Q. How does the electronic environment of silicon centers influence reactivity in cross-coupling reactions?
- Methodological Answer : The electron-donating ethoxy groups increase nucleophilicity at silicon, favoring transmetalation in Stille or Suzuki couplings. Computational studies (DFT) can model charge distribution, while X-ray crystallography of intermediates validates bonding geometries. Compare with tetraethoxysilane derivatives to isolate steric effects .
Q. What are the challenges in quantifying decomposition products under high-temperature conditions?
- Methodological Answer : Pyrolysis-GC-MS (300–400°C) identifies volatile byproducts (e.g., ethylene, silanols). Mitigate column adsorption by derivatizing silanols with trimethylsilylating agents. For non-volatile residues, use thermogravimetric analysis (TGA) coupled with FTIR to track Si-O network formation .
Properties
IUPAC Name |
ethenyl-[ethenyl(diethoxy)silyl]oxy-diethoxysilane | |
---|---|---|
Source | PubChem | |
URL | https://pubchem.ncbi.nlm.nih.gov | |
Description | Data deposited in or computed by PubChem | |
InChI |
InChI=1S/C12H26O5Si2/c1-7-13-18(11-5,14-8-2)17-19(12-6,15-9-3)16-10-4/h11-12H,5-10H2,1-4H3 | |
Source | PubChem | |
URL | https://pubchem.ncbi.nlm.nih.gov | |
Description | Data deposited in or computed by PubChem | |
InChI Key |
MZAYYDBNSRGYGH-UHFFFAOYSA-N | |
Source | PubChem | |
URL | https://pubchem.ncbi.nlm.nih.gov | |
Description | Data deposited in or computed by PubChem | |
Canonical SMILES |
CCO[Si](C=C)(OCC)O[Si](C=C)(OCC)OCC | |
Source | PubChem | |
URL | https://pubchem.ncbi.nlm.nih.gov | |
Description | Data deposited in or computed by PubChem | |
Molecular Formula |
C12H26O5Si2 | |
Source | PubChem | |
URL | https://pubchem.ncbi.nlm.nih.gov | |
Description | Data deposited in or computed by PubChem | |
DSSTOX Substance ID |
DTXSID80374458 | |
Record name | 1,3-DIVINYLTETRAETHOXYDISILOXANE | |
Source | EPA DSSTox | |
URL | https://comptox.epa.gov/dashboard/DTXSID80374458 | |
Description | DSSTox provides a high quality public chemistry resource for supporting improved predictive toxicology. | |
Molecular Weight |
306.50 g/mol | |
Source | PubChem | |
URL | https://pubchem.ncbi.nlm.nih.gov | |
Description | Data deposited in or computed by PubChem | |
CAS No. |
3682-26-6 | |
Record name | 1,3-DIVINYLTETRAETHOXYDISILOXANE | |
Source | EPA DSSTox | |
URL | https://comptox.epa.gov/dashboard/DTXSID80374458 | |
Description | DSSTox provides a high quality public chemistry resource for supporting improved predictive toxicology. | |
Disclaimer and Information on In-Vitro Research Products
Please be aware that all articles and product information presented on BenchChem are intended solely for informational purposes. The products available for purchase on BenchChem are specifically designed for in-vitro studies, which are conducted outside of living organisms. In-vitro studies, derived from the Latin term "in glass," involve experiments performed in controlled laboratory settings using cells or tissues. It is important to note that these products are not categorized as medicines or drugs, and they have not received approval from the FDA for the prevention, treatment, or cure of any medical condition, ailment, or disease. We must emphasize that any form of bodily introduction of these products into humans or animals is strictly prohibited by law. It is essential to adhere to these guidelines to ensure compliance with legal and ethical standards in research and experimentation.