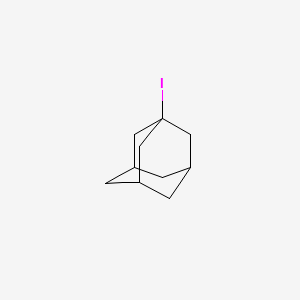
1-Iodoadamantane
Overview
Description
Adamantyl iodide, also known as 1-iodoadamantane, is an organoiodine compound derived from adamantane. Adamantane is a polycyclic hydrocarbon with a diamond-like structure, known for its high stability and unique properties. Adamantyl iodide is a versatile compound used in various chemical reactions and has significant applications in scientific research.
Mechanism of Action
Target of Action
1-Iodoadamantane is a haloadamantane It is known to be used as an iodine atom donor for probing the intermediacy of radical to investigate the chemistry of highly reactive, strained systems such as propellane .
Mode of Action
The mode of action of this compound involves its interaction with its targets. For instance, the voltammetric reduction of this compound at a silver cathode in tetrahydrofuran (THF) and acetonitrile (ACN) is reported to involve a single electron forming a mixture of monomeric and dimeric products .
Biochemical Pathways
It is known that this compound undergoes a photostimulated reaction with the enolate anion of acetone, acetophenone, and propiophenone to give adamantane and substitution products .
Pharmacokinetics
It is known that this compound is a solid compound with a molecular weight of 26213 .
Result of Action
The result of the action of this compound involves the formation of various products. For instance, the photoinduced reaction of this compound in DMSO is reported to afford substitution products on C3, C6, and C8, 1-adamantanol, 1-adamantyl 2-naphthyl ether, and adamantane .
Action Environment
The action environment can influence the efficacy and stability of this compound. For instance, β-Cyclodextrin (β-CD), a naturally occurring host, strongly binds adamantane and its derivatives. Based on this binding, a host–guest inclusion complex of β-CD with adamantane was prepared, and the included adamantane was selectively iodinated to this compound by iodoform .
Biochemical Analysis
Biochemical Properties
1-Iodoadamantane plays a significant role in biochemical reactions, particularly in the context of its interactions with enzymes and other biomolecules. It is known to undergo photostimulated reactions with various anions, such as the enolate anion of acetone, acetophenone, and propiophenone, resulting in the formation of substitution products . Additionally, this compound can act as an iodine atom donor in radical reactions, which is useful for probing the intermediacy of radicals in highly reactive systems . The compound’s interactions with enzymes and proteins are primarily driven by its ability to participate in electron transfer processes and form stable complexes with biomolecules.
Cellular Effects
This compound has been shown to influence various cellular processes, including cell signaling pathways, gene expression, and cellular metabolism. The compound’s ability to interact with cellular components, such as proteins and enzymes, can lead to alterations in cell function. For example, the photostimulated reaction of this compound with the phthalimide anion results in the formation of adamantane derivatives, which can affect cellular signaling pathways . Additionally, the compound’s interactions with enzymes involved in metabolic processes can lead to changes in cellular metabolism and gene expression.
Molecular Mechanism
The molecular mechanism of this compound’s action involves its ability to participate in electron transfer processes and form stable complexes with biomolecules. The compound undergoes voltammetric reduction at a silver cathode, resulting in the formation of monomeric and dimeric products . This reduction process is driven by a single electron transfer, which highlights the compound’s potential as an electron donor in biochemical reactions. Additionally, this compound’s interactions with enzymes and proteins can lead to enzyme inhibition or activation, depending on the nature of the interaction.
Temporal Effects in Laboratory Settings
In laboratory settings, the effects of this compound can change over time due to factors such as stability, degradation, and long-term effects on cellular function. The compound is known to be stable under certain conditions but can degrade when exposed to light and moisture . Long-term studies have shown that this compound can have sustained effects on cellular function, particularly in the context of its interactions with enzymes and proteins involved in metabolic processes.
Dosage Effects in Animal Models
The effects of this compound in animal models vary with different dosages. At lower doses, the compound can act as a modulator of cellular processes, while at higher doses, it may exhibit toxic or adverse effects. Studies have shown that the compound’s interactions with enzymes and proteins can lead to threshold effects, where a certain dosage is required to elicit a measurable response . Additionally, high doses of this compound can result in toxic effects, such as enzyme inhibition and disruption of cellular metabolism.
Metabolic Pathways
This compound is involved in various metabolic pathways, particularly those related to its interactions with enzymes and cofactors. The compound can undergo reduction and substitution reactions, which are catalyzed by enzymes such as SmI2 in the presence of hexamethylphosphoramide (HMPA) and water . These reactions can lead to changes in metabolic flux and metabolite levels, highlighting the compound’s role in modulating metabolic pathways.
Transport and Distribution
Within cells and tissues, this compound is transported and distributed through interactions with transporters and binding proteins. The compound’s lipophilic nature allows it to readily diffuse across cell membranes and accumulate in specific cellular compartments . Additionally, this compound can form complexes with binding proteins, which facilitate its transport and distribution within cells and tissues.
Subcellular Localization
The subcellular localization of this compound is influenced by its interactions with targeting signals and post-translational modifications. The compound can be directed to specific compartments or organelles within the cell, where it exerts its effects on cellular function . For example, this compound’s interactions with enzymes and proteins involved in metabolic processes can lead to its localization in organelles such as mitochondria and the endoplasmic reticulum.
Preparation Methods
Synthetic Routes and Reaction Conditions
Adamantyl iodide can be synthesized through several methods. One common method involves the reaction of adamantane with iodine in the presence of an oxidizing agent. This process typically requires specific reaction conditions, such as elevated temperatures and the presence of a catalyst. Another method involves the photostimulated reaction of adamantane with iodine, which can produce adamantyl iodide under controlled light exposure .
Industrial Production Methods
In industrial settings, the production of adamantyl iodide often involves large-scale reactions using optimized conditions to ensure high yields and purity. The use of continuous flow reactors and advanced purification techniques can enhance the efficiency of the production process.
Chemical Reactions Analysis
Types of Reactions
Adamantyl iodide undergoes various chemical reactions, including:
Substitution Reactions: Adamantyl iodide can participate in nucleophilic substitution reactions, where the iodine atom is replaced by other nucleophiles.
Oxidation and Reduction: Although less common, adamantyl iodide can undergo oxidation and reduction reactions under specific conditions.
Radical Reactions: Adamantyl iodide can be used as an iodine atom donor in radical reactions, which are useful for investigating the chemistry of highly reactive systems.
Common Reagents and Conditions
Common reagents used in reactions with adamantyl iodide include nucleophiles such as amines, alcohols, and thiols. Reaction conditions often involve the use of solvents like dichloromethane or acetonitrile, and catalysts such as Lewis acids or bases.
Major Products Formed
The major products formed from reactions involving adamantyl iodide depend on the specific reagents and conditions used. For example, nucleophilic substitution reactions with amines can produce adamantyl amines, while radical reactions can yield various substituted adamantane derivatives .
Scientific Research Applications
Comparison with Similar Compounds
Adamantyl iodide can be compared with other similar compounds, such as:
Adamantyl bromide: Similar to adamantyl iodide, adamantyl bromide is used in nucleophilic substitution reactions.
Adamantyl chloride: Adamantyl chloride is another related compound, but it is less reactive than adamantyl iodide.
The unique properties of adamantyl iodide, such as its high reactivity and stability, make it a valuable compound in various chemical and industrial applications.
Properties
IUPAC Name |
1-iodoadamantane | |
---|---|---|
Source | PubChem | |
URL | https://pubchem.ncbi.nlm.nih.gov | |
Description | Data deposited in or computed by PubChem | |
InChI |
InChI=1S/C10H15I/c11-10-4-7-1-8(5-10)3-9(2-7)6-10/h7-9H,1-6H2 | |
Source | PubChem | |
URL | https://pubchem.ncbi.nlm.nih.gov | |
Description | Data deposited in or computed by PubChem | |
InChI Key |
PXVOATXCSSPUEM-UHFFFAOYSA-N | |
Source | PubChem | |
URL | https://pubchem.ncbi.nlm.nih.gov | |
Description | Data deposited in or computed by PubChem | |
Canonical SMILES |
C1C2CC3CC1CC(C2)(C3)I | |
Source | PubChem | |
URL | https://pubchem.ncbi.nlm.nih.gov | |
Description | Data deposited in or computed by PubChem | |
Molecular Formula |
C10H15I | |
Source | PubChem | |
URL | https://pubchem.ncbi.nlm.nih.gov | |
Description | Data deposited in or computed by PubChem | |
DSSTOX Substance ID |
DTXSID00227619 | |
Record name | 1-Iodoadamantane | |
Source | EPA DSSTox | |
URL | https://comptox.epa.gov/dashboard/DTXSID00227619 | |
Description | DSSTox provides a high quality public chemistry resource for supporting improved predictive toxicology. | |
Molecular Weight |
262.13 g/mol | |
Source | PubChem | |
URL | https://pubchem.ncbi.nlm.nih.gov | |
Description | Data deposited in or computed by PubChem | |
CAS No. |
768-93-4 | |
Record name | 1-Iodoadamantane | |
Source | CAS Common Chemistry | |
URL | https://commonchemistry.cas.org/detail?cas_rn=768-93-4 | |
Description | CAS Common Chemistry is an open community resource for accessing chemical information. Nearly 500,000 chemical substances from CAS REGISTRY cover areas of community interest, including common and frequently regulated chemicals, and those relevant to high school and undergraduate chemistry classes. This chemical information, curated by our expert scientists, is provided in alignment with our mission as a division of the American Chemical Society. | |
Explanation | The data from CAS Common Chemistry is provided under a CC-BY-NC 4.0 license, unless otherwise stated. | |
Record name | 1-Iodoadamantane | |
Source | ChemIDplus | |
URL | https://pubchem.ncbi.nlm.nih.gov/substance/?source=chemidplus&sourceid=0000768934 | |
Description | ChemIDplus is a free, web search system that provides access to the structure and nomenclature authority files used for the identification of chemical substances cited in National Library of Medicine (NLM) databases, including the TOXNET system. | |
Record name | 1-Iodoadamantane | |
Source | DTP/NCI | |
URL | https://dtp.cancer.gov/dtpstandard/servlet/dwindex?searchtype=NSC&outputformat=html&searchlist=169440 | |
Description | The NCI Development Therapeutics Program (DTP) provides services and resources to the academic and private-sector research communities worldwide to facilitate the discovery and development of new cancer therapeutic agents. | |
Explanation | Unless otherwise indicated, all text within NCI products is free of copyright and may be reused without our permission. Credit the National Cancer Institute as the source. | |
Record name | 1-Iodoadamantane | |
Source | EPA DSSTox | |
URL | https://comptox.epa.gov/dashboard/DTXSID00227619 | |
Description | DSSTox provides a high quality public chemistry resource for supporting improved predictive toxicology. | |
Retrosynthesis Analysis
AI-Powered Synthesis Planning: Our tool employs the Template_relevance Pistachio, Template_relevance Bkms_metabolic, Template_relevance Pistachio_ringbreaker, Template_relevance Reaxys, Template_relevance Reaxys_biocatalysis model, leveraging a vast database of chemical reactions to predict feasible synthetic routes.
One-Step Synthesis Focus: Specifically designed for one-step synthesis, it provides concise and direct routes for your target compounds, streamlining the synthesis process.
Accurate Predictions: Utilizing the extensive PISTACHIO, BKMS_METABOLIC, PISTACHIO_RINGBREAKER, REAXYS, REAXYS_BIOCATALYSIS database, our tool offers high-accuracy predictions, reflecting the latest in chemical research and data.
Strategy Settings
Precursor scoring | Relevance Heuristic |
---|---|
Min. plausibility | 0.01 |
Model | Template_relevance |
Template Set | Pistachio/Bkms_metabolic/Pistachio_ringbreaker/Reaxys/Reaxys_biocatalysis |
Top-N result to add to graph | 6 |
Feasible Synthetic Routes
Disclaimer and Information on In-Vitro Research Products
Please be aware that all articles and product information presented on BenchChem are intended solely for informational purposes. The products available for purchase on BenchChem are specifically designed for in-vitro studies, which are conducted outside of living organisms. In-vitro studies, derived from the Latin term "in glass," involve experiments performed in controlled laboratory settings using cells or tissues. It is important to note that these products are not categorized as medicines or drugs, and they have not received approval from the FDA for the prevention, treatment, or cure of any medical condition, ailment, or disease. We must emphasize that any form of bodily introduction of these products into humans or animals is strictly prohibited by law. It is essential to adhere to these guidelines to ensure compliance with legal and ethical standards in research and experimentation.