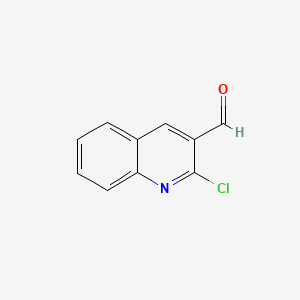
2-Chloroquinoline-3-carbaldehyde
Overview
Description
2-Chloroquinoline-3-carbaldehyde is a chemical compound that has attracted much attention due to its considerable biological and pharmacological activities . It is used as a precursor and building block for the synthesis of a wide range of heterocyclic systems and potent antibiotics for microbial and cancer treatment .
Synthesis Analysis
The synthesis of 2-Chloroquinoline-3-carbaldehydes involves the Vilsmeier-Haack reaction . This reaction involves the preparation of 2-Chloroquinoline-3-carbaldehydes and 2-chloro-4-methyl-quinoline-3-carbaldehyde from acetanilide and acetoacetanilide .Molecular Structure Analysis
The molecular structure of 2-Chloroquinoline-3-carbaldehyde is based on the quinoline ring system . The quinoline ring system is a basic skeleton of many naturally occurring compounds .Chemical Reactions Analysis
2-Chloroquinoline-3-carbaldehydes undergo various chemical reactions due to the presence of two active moieties, chloro- and aldehyde functions . These reactions include substitution reactions, reactions with primary amines to produce Schiff’s bases, and reactions with substituted isothiocyanates and α-halocarbonyl compounds .Physical And Chemical Properties Analysis
2-Chloroquinoline-3-carbaldehyde appears as a slightly pale yellow to yellow-green crystal or powder . It has a CAS Number of 73568-25-9 .Scientific Research Applications
Synthesis of Quinoline Ring Systems
2-Chloroquinoline-3-carbaldehyde: is pivotal in the synthesis of quinoline ring systems, which are a fundamental scaffold in many pharmacologically active compounds . The versatility of the quinoline core allows for the construction of diverse fused or binary quinoline-containing heterocyclic systems. These systems are often pursued for their potential biological activities.
Antimicrobial Applications
Compounds derived from 2-Chloroquinoline-3-carbaldehyde have demonstrated significant antimicrobial properties . This includes activity against a range of bacteria and fungi, making these derivatives valuable in the development of new antimicrobial agents.
Antimalarial Activity
The structural analogs of 2-Chloroquinoline-3-carbaldehyde have been explored for their antimalarial properties . Given the ongoing challenge of drug-resistant malaria strains, these compounds offer a promising avenue for new therapeutic options.
Anti-inflammatory Properties
Derivatives of 2-Chloroquinoline-3-carbaldehyde have shown anti-inflammatory activity , which is crucial in the treatment of chronic inflammatory diseases. The ability to modulate inflammatory responses makes these compounds candidates for further drug development.
Antitumor and Anticancer Research
Research has indicated that certain 2-Chloroquinoline-3-carbaldehyde derivatives possess antitumor and anticancer activities . These compounds can be designed to target specific pathways involved in cancer progression, offering potential for targeted cancer therapies.
Anti-parasitic Applications
The chemical versatility of 2-Chloroquinoline-3-carbaldehyde allows for the creation of compounds with anti-parasitic effects . This is particularly relevant for the treatment of diseases caused by parasitic infections, which remain a significant health burden in many parts of the world.
Safety and Hazards
Future Directions
The future directions for 2-Chloroquinoline-3-carbaldehyde involve further exploration of its chemistry and applications . Recent research data highlights the synthesis of quinoline ring systems and reactions adopted to construct fused or binary quinoline-cord heterocyclic systems . The biological evaluation and the synthetic applications of the target compounds are areas of ongoing research .
Mechanism of Action
Target of Action
Quinolines, the class of compounds to which 2-chloroquinoline-3-carbaldehyde belongs, are known to exhibit various biological and pharmaceutical activities .
Mode of Action
The mode of action of 2-Chloroquinoline-3-carbaldehyde involves its interaction with its targets, leading to various changes. The specific interactions and changes depend on the target and the biological context .
Biochemical Pathways
2-Chloroquinoline-3-carbaldehyde affects various biochemical pathways. The specific pathways and their downstream effects are dependent on the biological context and the targets of the compound .
Result of Action
The molecular and cellular effects of 2-Chloroquinoline-3-carbaldehyde’s action depend on its targets and the biological context .
Action Environment
Environmental factors can influence the action, efficacy, and stability of 2-Chloroquinoline-3-carbaldehyde. These factors can include the biological context, the presence of other compounds, and physical conditions such as temperature and pH .
properties
IUPAC Name |
2-chloroquinoline-3-carbaldehyde | |
---|---|---|
Source | PubChem | |
URL | https://pubchem.ncbi.nlm.nih.gov | |
Description | Data deposited in or computed by PubChem | |
InChI |
InChI=1S/C10H6ClNO/c11-10-8(6-13)5-7-3-1-2-4-9(7)12-10/h1-6H | |
Source | PubChem | |
URL | https://pubchem.ncbi.nlm.nih.gov | |
Description | Data deposited in or computed by PubChem | |
InChI Key |
SDKQWXCBSNMYBN-UHFFFAOYSA-N | |
Source | PubChem | |
URL | https://pubchem.ncbi.nlm.nih.gov | |
Description | Data deposited in or computed by PubChem | |
Canonical SMILES |
C1=CC=C2C(=C1)C=C(C(=N2)Cl)C=O | |
Source | PubChem | |
URL | https://pubchem.ncbi.nlm.nih.gov | |
Description | Data deposited in or computed by PubChem | |
Molecular Formula |
C10H6ClNO | |
Source | PubChem | |
URL | https://pubchem.ncbi.nlm.nih.gov | |
Description | Data deposited in or computed by PubChem | |
DSSTOX Substance ID |
DTXSID80351108 | |
Record name | 2-chloroquinoline-3-carbaldehyde | |
Source | EPA DSSTox | |
URL | https://comptox.epa.gov/dashboard/DTXSID80351108 | |
Description | DSSTox provides a high quality public chemistry resource for supporting improved predictive toxicology. | |
Molecular Weight |
191.61 g/mol | |
Source | PubChem | |
URL | https://pubchem.ncbi.nlm.nih.gov | |
Description | Data deposited in or computed by PubChem | |
Product Name |
2-Chloroquinoline-3-carbaldehyde | |
CAS RN |
73568-25-9 | |
Record name | 2-chloroquinoline-3-carbaldehyde | |
Source | EPA DSSTox | |
URL | https://comptox.epa.gov/dashboard/DTXSID80351108 | |
Description | DSSTox provides a high quality public chemistry resource for supporting improved predictive toxicology. | |
Record name | 2-Chloro-3-quinolinecarboxaldehyde | |
Source | European Chemicals Agency (ECHA) | |
URL | https://echa.europa.eu/information-on-chemicals | |
Description | The European Chemicals Agency (ECHA) is an agency of the European Union which is the driving force among regulatory authorities in implementing the EU's groundbreaking chemicals legislation for the benefit of human health and the environment as well as for innovation and competitiveness. | |
Explanation | Use of the information, documents and data from the ECHA website is subject to the terms and conditions of this Legal Notice, and subject to other binding limitations provided for under applicable law, the information, documents and data made available on the ECHA website may be reproduced, distributed and/or used, totally or in part, for non-commercial purposes provided that ECHA is acknowledged as the source: "Source: European Chemicals Agency, http://echa.europa.eu/". Such acknowledgement must be included in each copy of the material. ECHA permits and encourages organisations and individuals to create links to the ECHA website under the following cumulative conditions: Links can only be made to webpages that provide a link to the Legal Notice page. | |
Synthesis routes and methods I
Procedure details
Synthesis routes and methods II
Procedure details
Synthesis routes and methods III
Procedure details
Retrosynthesis Analysis
AI-Powered Synthesis Planning: Our tool employs the Template_relevance Pistachio, Template_relevance Bkms_metabolic, Template_relevance Pistachio_ringbreaker, Template_relevance Reaxys, Template_relevance Reaxys_biocatalysis model, leveraging a vast database of chemical reactions to predict feasible synthetic routes.
One-Step Synthesis Focus: Specifically designed for one-step synthesis, it provides concise and direct routes for your target compounds, streamlining the synthesis process.
Accurate Predictions: Utilizing the extensive PISTACHIO, BKMS_METABOLIC, PISTACHIO_RINGBREAKER, REAXYS, REAXYS_BIOCATALYSIS database, our tool offers high-accuracy predictions, reflecting the latest in chemical research and data.
Strategy Settings
Precursor scoring | Relevance Heuristic |
---|---|
Min. plausibility | 0.01 |
Model | Template_relevance |
Template Set | Pistachio/Bkms_metabolic/Pistachio_ringbreaker/Reaxys/Reaxys_biocatalysis |
Top-N result to add to graph | 6 |
Feasible Synthetic Routes
Q & A
Q1: What is the molecular formula and weight of 2-Chloroquinoline-3-carbaldehyde?
A1: The molecular formula is C10H6ClNO, and the molecular weight is 191.62 g/mol.
Q2: Which spectroscopic techniques are commonly employed to characterize 2-Chloroquinoline-3-carbaldehyde and its derivatives?
A2: Researchers frequently utilize FTIR, 1H NMR, 13C NMR, and mass spectrometry to confirm the structure of 2-Chloroquinoline-3-carbaldehyde and its synthesized derivatives. , , These techniques provide valuable information about functional groups, proton and carbon environments, and molecular mass, respectively.
Q3: What is the common starting material for synthesizing 2-Chloroquinoline-3-carbaldehyde?
A3: The synthesis typically starts with readily available acetanilides, which undergo Vilsmeier-Haack formylation to yield the desired 2-Chloroquinoline-3-carbaldehyde. , This well-established reaction provides a straightforward route to this key intermediate.
Q4: How does phosphorus pentachloride act as an alternative chlorinating agent in synthesizing 2-Chloroquinoline-3-carbaldehydes?
A4: Employing phosphorus pentachloride (PCl5) as a chlorinating agent instead of phosphoryl chloride (POCl3) in the Vilsmeier-Haack reaction offers an alternative route, particularly beneficial for activated acetanilides. This modified procedure can lead to good yields of 2-Chloroquinoline-3-carbaldehydes.
Q5: How does 2-Chloroquinoline-3-carbaldehyde react with stabilized methylenetriphenylphosphoranes?
A5: The reaction of 2-Chloroquinoline-3-carbaldehyde with stabilized phosphonium ylides, a classic Wittig reaction, results in a mixture of E and Z isomers of the corresponding olefins. This reaction allows for the introduction of various substituents onto the quinoline core, expanding the diversity of accessible compounds.
Q6: Can you elaborate on the reaction of 2-Chloroquinoline-3-carbaldehyde with isocyanides?
A6: 2-Chloroquinoline-3-carbaldehyde displays interesting reactivity towards isocyanides, leading to diverse quinoline derivatives through multicomponent reactions. In the presence of a palladium catalyst, it can undergo a domino reaction with one or two equivalents of isocyanides, forging new C-C and C-N bonds. Moreover, incorporating amines in these reactions enables the formation of complex heterocyclic systems, highlighting the versatility of this approach. ,
Q7: How is 2-Chloroquinoline-3-carbaldehyde employed in constructing fused heterocyclic systems?
A7: The compound serves as a crucial building block for synthesizing diverse fused heterocyclic systems, often achieved through multicomponent reactions. For instance, reacting it with dimedone and ammonium acetate under mild conditions yields 3,3,6,6-tetramethyl-9-quinolyl-2,4,5,7,9,10-hexahydroacridine-1,8(2H,5H)-dione derivatives. These reactions showcase the efficiency of using 2-Chloroquinoline-3-carbaldehyde in accessing complex molecular architectures.
Q8: What are some eco-friendly approaches to synthesizing 2-Chloroquinoline-3-carbaldehydes and their derivatives?
A8: Several green chemistry principles have been implemented to minimize the environmental footprint of 2-Chloroquinoline-3-carbaldehyde synthesis and subsequent reactions. Microwave-assisted synthesis has proven highly effective in accelerating reaction rates and improving yields for various quinoline derivatives. , , Additionally, using water as a solvent and exploring solvent-free conditions, such as grinding, further contributes to more sustainable synthetic routes. These green chemistry practices align with the growing emphasis on environmentally responsible synthetic methodologies.
Q9: How can ionic liquids be utilized in reactions involving 2-Chloroquinoline-3-carbaldehyde?
A9: Ionic liquids have been successfully employed as catalysts in Knoevenagel condensations involving 2-Chloroquinoline-3-carbaldehyde and various active methylene compounds. This method offers advantages such as mild reaction conditions, short reaction times, excellent yields, and reduced environmental impact by avoiding harsh solvents.
Q10: Can you provide an example of a one-pot multicomponent reaction (MCR) utilizing 2-Chloroquinoline-3-carbaldehyde?
A10: A representative example is the synthesis of 3,3-dimethyl-3,4-dihydro-2H-dibenzo[b,g][1,8]-naphthyridin-1-one derivatives. This transformation involves a three-component reaction between 2-Chloroquinoline-3-carbaldehydes, cyclic hexanedione, and ammonium acetate in an aqueous medium. This MCR strategy offers high selectivity and good yields while adhering to green chemistry principles.
Q11: What are the potential biological applications of 2-Chloroquinoline-3-carbaldehyde derivatives?
A11: The diverse array of 2-Chloroquinoline-3-carbaldehyde derivatives exhibits a broad range of promising biological activities. Notably, these compounds have shown potential as antimicrobial agents, , , , antioxidants, , , and anti-inflammatory agents. Their ability to inhibit specific enzymes, such as AKT1, further highlights their potential in developing novel therapeutics.
Q12: Have there been studies on the DNA binding properties of 2-Chloroquinoline-3-carbaldehyde derivatives?
A12: Yes, research has explored the interaction of certain 2-Chloroquinoline-3-carbaldehyde derivatives with DNA. For example, compounds like 2-chloroquinoline-3-carbaldehyde [(2-hydroxy-1-naphthyl)methylene] hydrazone have shown interactions with calf thymus DNA (CT-DNA). These studies often utilize techniques like electronic spectroscopy, viscosity measurements, and thermal denaturation studies to characterize the binding affinity and mode of interaction.
Q13: How is computational chemistry applied in research involving 2-Chloroquinoline-3-carbaldehyde?
A13: Computational chemistry plays a significant role in understanding the properties and behavior of 2-Chloroquinoline-3-carbaldehyde and its derivatives. Molecular modeling studies, often employing docking simulations, help predict binding affinities and interactions with target proteins like PI3K. Additionally, researchers utilize quantitative structure-activity relationship (QSAR) models to correlate molecular structure with biological activity, enabling the design of more potent and selective compounds. ,
Q14: Can you elaborate on the use of DFT calculations in studying 2-Chloroquinoline-3-carbaldehyde reactions?
A14: Density Functional Theory (DFT) calculations have proven valuable in predicting the reactivity and regioselectivity of reactions involving 2-Chloroquinoline-3-carbaldehyde. For example, in the synthesis of 1,3-diazaheterocycle fused [1,2-a][1,8]naphthyridine derivatives, DFT calculations, combined with frontier molecular orbital (FMO) theory, enabled researchers to optimize reaction conditions and accurately predict the regiochemical outcome. This approach highlights the power of computational methods in guiding synthetic strategies and understanding reaction mechanisms.
Disclaimer and Information on In-Vitro Research Products
Please be aware that all articles and product information presented on BenchChem are intended solely for informational purposes. The products available for purchase on BenchChem are specifically designed for in-vitro studies, which are conducted outside of living organisms. In-vitro studies, derived from the Latin term "in glass," involve experiments performed in controlled laboratory settings using cells or tissues. It is important to note that these products are not categorized as medicines or drugs, and they have not received approval from the FDA for the prevention, treatment, or cure of any medical condition, ailment, or disease. We must emphasize that any form of bodily introduction of these products into humans or animals is strictly prohibited by law. It is essential to adhere to these guidelines to ensure compliance with legal and ethical standards in research and experimentation.