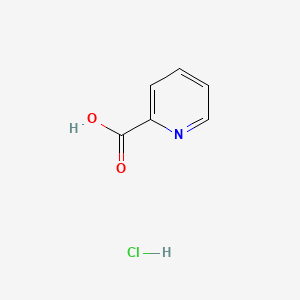
Picolinic Acid Hydrochloride
Overview
Description
Picolinic acid hydrochloride (PHCL; CAS 636-80-6) is the hydrochloride salt of picolinic acid (pyridine-2-carboxylic acid). It is a white to off-white crystalline solid with a molecular weight of 173.57 g/mol. PHCL is synthesized via the oxidation of α-picoline (2-methylpyridine) using potassium permanganate (KMnO₄) under acidic conditions, followed by hydrochloric acid treatment .
Key properties include:
- Crystallography: Orthorhombic crystal system (space group P21/c), confirmed by single-crystal X-ray diffraction .
- Optical Behavior: Exhibits nonlinear optical (NLO) activity, emitting green light (532 nm) under Nd:YAG laser irradiation (1064 nm) due to second harmonic generation .
- Physical Stability: High mechanical strength (Vickers hardness: ~75 HV) and thermal stability (decomposition >200°C) .
- Solubility: Soluble in polar solvents (ethanol, methanol) but insoluble in nonpolar solvents like chloroform .
PHCL is utilized in coordination chemistry, crystal engineering, and as a precursor for pharmaceuticals and ligands .
Preparation Methods
Oxidation of α-Picoline to Picolinic Acid
Potassium Permanganate Oxidation Method
This classical and widely used method involves the oxidation of α-picoline with potassium permanganate in an aqueous medium, followed by acidification and isolation of picolinic acid hydrochloride.
Procedure Summary:
- In a 5-liter three-necked flask equipped with a reflux condenser and stirrer, 50 g (0.54 mole) of α-picoline is mixed with 2500 ml of water.
- 90 g (0.57 mole) of potassium permanganate is added, and the mixture is heated on a steam bath until the purple color of permanganate nearly disappears (~1 hour).
- A second 90 g portion of potassium permanganate and 500 ml of water are added, and heating continues for 2–2.5 hours until the purple color is destroyed.
- The mixture is cooled, manganese oxides are filtered off and washed.
- The filtrate is concentrated under reduced pressure to 150–200 ml, filtered if necessary, then acidified with 65–70 ml of concentrated hydrochloric acid (sp. gr. 1.19).
- The acidified solution is evaporated to dryness under reduced pressure.
- The residue is refluxed with 95% ethanol, filtered, and the extraction repeated.
- Dry hydrogen chloride gas is passed into the combined ethanolic filtrates until crystallization begins.
- The solution is chilled to about 10°C, and hydrogen chloride addition continues until saturation.
- Crystals of this compound are collected by filtration and air-dried.
- Yield: 43–44 g (50–51%)
- Melting point: 228–230°C (initial), purified product melts at 210–212°C (230°C reported)
- Purification involves recrystallization from hot absolute ethanol followed by ether addition to remove potassium chloride impurities.
- The hydrochloride salt may contain residual potassium chloride, removable by recrystallization.
- The method is robust for laboratory-scale synthesis and provides good purity.
This method is documented in Organic Syntheses and is considered a benchmark for this compound preparation.
Alternative Synthetic Routes and Oxidation Methods
Hydrolysis of 2-Cyanopyridine
An alternative preparation of picolinic acid involves hydrolysis of 2-cyanopyridine under alkaline conditions:
- 2-Cyanopyridine is reacted with sodium hydroxide in deionized water at 50°C.
- The reaction mixture is refluxed for several hours (typically 4 hours).
- After completion, water is distilled off, and the product is isolated.
- The crude acid can be purified by extraction and chromatography.
This method yields pyridine-2-carboxylic acid (picolinic acid) which can subsequently be converted to the hydrochloride salt by treatment with hydrochloric acid and crystallization.
Catalytic Oxidation Using Nanocomposites
Recent research explores catalytic oxidation of alcohol derivatives to carboxylic acids, including picolinic acid, using Ag/ZnO/graphene nanocomposites with TBSCl in methanol-water mixtures. This method is more experimental and used for derivatives but indicates potential for mild, selective oxidation routes.
Conversion of Picolinic Acid to this compound
The formation of the hydrochloride salt is achieved by:
- Dissolving picolinic acid in ethanol or other suitable solvents.
- Passing dry hydrogen chloride gas into the solution until saturation and crystallization occur.
- Cooling the solution to enhance crystallization.
- Filtering and drying the resulting this compound crystals.
This step is crucial for obtaining the stable crystalline hydrochloride salt suitable for storage and further applications.
Comparative Data Table of Preparation Methods
Chemical Reactions Analysis
Amide Formation via Acid Chloride Intermediate
Picolinic acid hydrochloride serves as a precursor in synthesizing amides through acid chloride intermediates . When treated with thionyl chloride (SOCl₂), it forms picolinoyl chloride, which reacts with amines to yield substituted amides . Notably, this reaction can produce 4-chloro derivatives as byproducts due to nucleophilic aromatic substitution during acid chloride formation .
Example Reaction:
textPicolinic acid + SOCl₂ → Picolinoyl chloride Picolinoyl chloride + N-alkylaniline → N-alkyl-N-phenylpicolinamide + 4-chloro-N-alkyl-N-phenylpicolinamide
Key Findings:
-
Yields for mono-amides range from 31% to 54% , while chlorinated byproducts are isolated in 10%–13% yields .
-
X-ray crystallography reveals a preference for cis amide geometry, with aromatic groups positioned cis to each other .
Reduction
Hydrogenation of picolinic acid derivatives produces piperidine-2-carboxylic acid , a precursor to pharmaceuticals like mepivacaine . The hydrochloride form facilitates such reductions by stabilizing intermediates.
Oxidation
In the presence of potassium permanganate (KMnO₄) , picolinic acid undergoes oxidation. While specific data on the hydrochloride form is limited, analogous reactions yield 6-hydroxypicolinic acid .
Role in Fenton-like Reactions
Picolinic acid enhances Mn(II)-accelerated Fenton systems by promoting Fe(III)/Fe(II) redox cycling. In acidic conditions (pH 4.5–6.0), Mn(II) complexes with a ferric hydroperoxo intermediate (PICA-Feᴵᴵᴵ-OOH), enabling intramolecular electron transfer to generate Fe(II) and MnO₂⁺ . This cycle produces hydroxyl radicals (HO- ) and superoxide (O₂- ⁻), critical for pollutant degradation.
Mechanism Highlights:
-
Fe(III) Reduction: Mn(II) accelerates Fe(III) → Fe(II) conversion, sustaining HO- generation.
-
Degradation Efficiency: Achieves >90% removal of contaminants like atrazine under optimized conditions .
Chlorination Side Reactions
During acid chloride synthesis with SOCl₂, 4-chloropicolinoyl chloride forms as a side product. This occurs via chloride attack on the activated pyridine ring, particularly under prolonged reaction times .
Mitigation:
-
Chlorinated byproducts are separable via column chromatography .
-
No chlorination is observed in analogous reactions with pyridine-2,6-dicarboxylic acid .
Coordination Chemistry
This compound acts as a bidentate ligand , chelating metals like Cr(III), Zn(II), and Cu(II) through its pyridine nitrogen and carboxylate oxygen . These complexes are pivotal in catalysis and nutrient absorption.
Example Complexes:
Scientific Research Applications
Coordination Chemistry
PAH serves as a chelating agent , forming stable complexes with various metals such as zinc, manganese, copper, and iron. This property is pivotal in coordination chemistry for synthesizing metal complexes used in catalysis and material science.
Biological Roles
- Zinc Transport : PAH plays a crucial role in the transport of zinc ions within biological systems, which is essential for numerous enzymatic reactions and cellular functions.
- Neuroprotective Effects : It exhibits neuroprotective properties by modulating neurotransmitter systems and reducing oxidative stress.
Antiviral Activity
Recent studies have demonstrated that PAH possesses broad-spectrum antiviral properties, particularly against enveloped viruses such as SARS-CoV-2 and Influenza A virus. The mechanisms include:
- Inhibition of Viral Entry : PAH disrupts the fusion process between viral membranes and host cells, preventing infection .
- Preclinical Efficacy : In animal models, PAH has shown significant reductions in viral loads post-infection with SARS-CoV-2 .
Case Study 1: Antiviral Efficacy Against SARS-CoV-2
A study involving Syrian golden hamsters indicated that administration of PAH significantly reduced viral loads in respiratory tissues after SARS-CoV-2 infection. Treatment with PAH at concentrations of 1 mM and 2 mM resulted in a reduction of approximately one log10 in viral RNA levels within 72 hours post-infection.
Case Study 2: Immunomodulatory Effects
In macrophage studies under inflammatory conditions, PAH enhanced the production of chemokines MIP-1α and MIP-1β, which are vital for leukocyte recruitment to inflammation sites. This suggests its potential utility in managing inflammatory diseases.
Summary Table of Applications
Application Area | Specific Uses | Mechanism/Effect |
---|---|---|
Coordination Chemistry | Chelating agent for metals | Forms stable metal complexes |
Biological Research | Zinc transport, neuroprotection | Modulates enzymatic reactions |
Medicine | Antiviral treatment | Inhibits viral entry and membrane fusion |
Immunology | Enhances chemokine production | Aids in leukocyte recruitment during inflammation |
Mechanism of Action
Picolinic acid hydrochloride exerts its effects by binding to zinc finger proteins, altering their structures, and disrupting zinc binding. This inhibition affects viral replication and packaging, as well as normal cell homeostatic functions . The compound’s role in zinc transport is crucial for its immunomodulatory and anti-infective properties.
Comparison with Similar Compounds
Structural and Functional Analogues
3-Methylpicolinic Acid Hydrochloride (CAS 123811-72-3)
- Structure : Methyl substituent at the 3-position of the pyridine ring.
- Properties: Molecular weight: 173.6 g/mol (vs. 173.57 g/mol for PHCL). Steric effects reduce coordination flexibility compared to PHCL.
4-Chloro-2-Picolinic Acid Hydrochloride (CAS 1036648-06-2)
- Structure : Chlorine substituent at the 4-position.
- Properties :
Piperidine-2-Carboxylic Acid Hydrochloride (CAS 2133-33-7)
- Structure : Saturated six-membered ring (piperidine) instead of aromatic pyridine.
- Properties :
Coordination Chemistry Comparison
Mercury(II) Complexes
- PHCL-derived complexes exhibit solvent-dependent dimensionality. Aqueous solvents favor polymeric structures, while alcoholic solvents stabilize mononuclear species .
Palladium(II) Complexes
- PHCL derivatives (e.g., picolinamide ligands) form stable Pd(II) complexes for catalytic applications. In contrast, nicotinic acid (pyridine-3-carboxylic acid) complexes show lower stability due to less favorable N,O-chelation .
Physicochemical Properties
Compound | Melting Point (°C) | Solubility (Polar Solvents) | LD₅₀ (Mouse, Oral) | Key Applications |
---|---|---|---|---|
This compound | 162 (dec.) | High (ethanol, methanol) | 750 mg/kg | NLO crystals, ligands |
Picolinic Acid N-Oxide | 162 (dec.) | Moderate | N/A | Oxidizing agent |
3-Methylpicolinic Acid HCl | N/A | Moderate | N/A | Organic synthesis |
4-Chloro-2-Picolinic Acid | N/A | Low | N/A | Anticancer drug precursor |
Biological Activity
Picolinic acid hydrochloride (PAH) is a derivative of picolinic acid, a naturally occurring metabolite of tryptophan. It has gained attention for its diverse biological activities, particularly in the fields of virology, immunology, and microbiology. This article presents a comprehensive overview of the biological activity of this compound, supported by relevant data tables, case studies, and research findings.
Antiviral Properties
Picolinic acid has demonstrated broad-spectrum antiviral activity, particularly against enveloped viruses such as SARS-CoV-2 and influenza A virus (IAV). Research indicates that PAH inhibits viral entry by compromising membrane integrity and interfering with cellular endocytosis. In pre-clinical studies, PAH exhibited significant antiviral efficacy against these viruses in animal models, suggesting its potential as a therapeutic agent during viral pandemics .
- Membrane Fusion Inhibition : PAH disrupts the fusion process between viral and cellular membranes, preventing the entry of viruses into host cells.
- Endosomal Maturation : It affects endosome maturation, a critical step in the viral entry pathway .
Immunomodulatory Effects
Picolinic acid also plays a role in modulating immune responses. It has been shown to enhance the activity of macrophages in inflammatory conditions by augmenting the production of reactive nitrogen intermediates. This effect is synergistic with interferon-gamma (IFN-γ), which is crucial for activating macrophage functions .
Table 1: Summary of Immunomodulatory Effects
Effect | Mechanism | Reference |
---|---|---|
Augmentation of NO production | Synergistic action with IFN-γ | |
Induction of MIP-1α/β | Involved in Th1 responses | |
Zinc transport facilitation | Enhances immune function |
Antimicrobial Activity
In addition to its antiviral properties, picolinic acid exhibits antimicrobial activity against various pathogens. It has been found effective against bacteria such as Staphylococcus aureus and Pseudomonas aeruginosa at specific concentrations. Studies have indicated low cytotoxicity at therapeutic doses, making it a candidate for food preservation and therapeutic applications .
Table 2: Antimicrobial Efficacy
Pathogen | Minimum Inhibitory Concentration (MIC) | Reference |
---|---|---|
Staphylococcus aureus | 0.78 mg/mL (pH 5.0) | |
Pseudomonas aeruginosa | 0.39 mg/mL (pH 5.0) | |
Candida albicans | 0.39 mg/mL (pH 5.0) |
Case Study 1: Antiviral Efficacy Against SARS-CoV-2
A study conducted on Syrian golden hamsters demonstrated that administration of picolinic acid significantly reduced viral loads in respiratory tissues following infection with SARS-CoV-2. The results indicated a reduction of approximately one log10 in viral RNA levels within 72 hours post-infection when treated with PAH at concentrations of 1 mM and 2 mM .
Case Study 2: Immunomodulation in Inflammatory Conditions
In another investigation involving macrophages under inflammatory conditions, picolinic acid was shown to enhance the production of MIP-1α and MIP-1β chemokines. This effect was crucial for leukocyte recruitment to sites of inflammation, highlighting PAH's potential role in managing inflammatory diseases .
Q & A
Basic Research Questions
Q. What are the optimal synthetic methods for preparing picolinic acid hydrochloride derivatives, and how can reaction conditions be optimized?
this compound derivatives are commonly synthesized via coupling reactions. For example, picolinic acid reacts with amines (e.g., 2-chloroethanamine hydrochloride) in acetonitrile using activating agents like N,N'-dicyclohexylcarbodiimide (DCC) and hydroxybenzotriazole (HOBt), with triethylamine (TEA) as a base . Optimization involves adjusting solvent polarity, stoichiometry, and reaction time to maximize yield. Kinetic studies and thin-layer chromatography (TLC) monitoring are recommended for intermediate validation.
Q. What safety protocols are critical when handling this compound in laboratory settings?
- Personal Protective Equipment (PPE): Wear nitrile gloves, lab coats, and safety goggles to avoid skin/eye contact, which can cause irritation .
- Spill Management: Use inert absorbents (e.g., vermiculite) and avoid water jets to prevent dispersion. Contaminated materials must be treated as biohazard waste .
- Ventilation: Work in a fume hood to mitigate inhalation risks, as respiratory irritation is possible .
Q. Which analytical techniques are most effective for quantifying this compound and its metabolites?
- UV-Vis Spectrophotometry: The Bradford assay (protein-dye binding principle) can quantify microgram-level protein-picolinate complexes, though interference from detergents requires validation .
- LC-MS/MS: Electrospray ionization (ESI) in positive ion mode with a C18 column and methanol/water mobile phase provides high sensitivity for metabolic profiling (e.g., detecting hemiacetal forms) .
- Solid-State NMR: Resolves proton environments in crystalline derivatives, such as hydroxyl groups in hemiacetal structures .
Advanced Research Questions
Q. How does this compound act as a catalyst in oxidation reactions, and what factors influence its mechanistic pathway?
Picolinic acid accelerates chromic acid (Cr(VI)) oxidations of alcohols via a termolecular complex involving Cr(VI), picolinic acid, and the substrate. Key mechanistic insights:
- Acidity Dependence: At low [H⁺], the reaction is second-order in H⁺ and rate-limited by complex formation. At high [H⁺], decomposition of the complex becomes rate-limiting, with a primary deuterium isotope effect (k_H/k_D ≈ 6) .
- Steric Effects: 6-substituted picolinic acids show reduced activity due to hindered octahedral coordination around Cr(VI) .
- Table 1: Kinetic parameters under varying acidities:
[H⁺] (M) | Rate Law | Activation Energy (kJ/mol) |
---|---|---|
0.1 | k[Cr][PA][H⁺]² | 58 ± 3 |
1.0 | k[Cr][PA][H⁺]⁰ | 72 ± 4 |
Q. What crystallographic challenges arise when resolving hydrogen atom positions in this compound derivatives?
X-ray diffraction at 100 K reveals shared hydrogen atoms between carboxylate/pyridinic nitrogen moieties, appearing as split occupancies (e.g., 0.5 occupancy per site). Fourier difference maps and DFT calculations are critical to validate these positions, as conventional refinement may misassign electron density . For example, in pyridoxal hydrochloride, solid-state NMR confirmed hemiacetal protonation states that X-ray alone could not resolve .
Q. How do structural modifications (e.g., chloro or cyclopropyl substituents) alter the bioactivity of this compound derivatives?
- 4-Chloro-6-cyclopropylpicolinic acid: The chloro group enhances electrophilicity, improving binding to metalloenzymes, while the cyclopropyl moiety increases lipophilicity, enhancing membrane permeability .
- Regorafenib derivative (4-chloro-2-picolinic acid): Chlorination at the 2-position stabilizes π-stacking interactions with kinase active sites, as shown in molecular docking studies .
- Methodological Tip: Use Hammett constants (σ) to predict electronic effects and logP calculations to optimize bioavailability.
Properties
IUPAC Name |
pyridine-2-carboxylic acid;hydrochloride | |
---|---|---|
Source | PubChem | |
URL | https://pubchem.ncbi.nlm.nih.gov | |
Description | Data deposited in or computed by PubChem | |
InChI |
InChI=1S/C6H5NO2.ClH/c8-6(9)5-3-1-2-4-7-5;/h1-4H,(H,8,9);1H | |
Source | PubChem | |
URL | https://pubchem.ncbi.nlm.nih.gov | |
Description | Data deposited in or computed by PubChem | |
InChI Key |
HFAFXVOPGDBAOK-UHFFFAOYSA-N | |
Source | PubChem | |
URL | https://pubchem.ncbi.nlm.nih.gov | |
Description | Data deposited in or computed by PubChem | |
Canonical SMILES |
C1=CC=NC(=C1)C(=O)O.Cl | |
Source | PubChem | |
URL | https://pubchem.ncbi.nlm.nih.gov | |
Description | Data deposited in or computed by PubChem | |
Molecular Formula |
C6H6ClNO2 | |
Source | PubChem | |
URL | https://pubchem.ncbi.nlm.nih.gov | |
Description | Data deposited in or computed by PubChem | |
Related CAS |
98-98-6 (Parent) | |
Record name | 2-Pyridinecarboxylic acid, hydrochloride (1:1) | |
Source | ChemIDplus | |
URL | https://pubchem.ncbi.nlm.nih.gov/substance/?source=chemidplus&sourceid=0000636806 | |
Description | ChemIDplus is a free, web search system that provides access to the structure and nomenclature authority files used for the identification of chemical substances cited in National Library of Medicine (NLM) databases, including the TOXNET system. | |
DSSTOX Substance ID |
DTXSID7060912 | |
Record name | Picolinic acid hydrochloride | |
Source | EPA DSSTox | |
URL | https://comptox.epa.gov/dashboard/DTXSID7060912 | |
Description | DSSTox provides a high quality public chemistry resource for supporting improved predictive toxicology. | |
Molecular Weight |
159.57 g/mol | |
Source | PubChem | |
URL | https://pubchem.ncbi.nlm.nih.gov | |
Description | Data deposited in or computed by PubChem | |
CAS No. |
636-80-6 | |
Record name | 2-Pyridinecarboxylic acid, hydrochloride (1:1) | |
Source | CAS Common Chemistry | |
URL | https://commonchemistry.cas.org/detail?cas_rn=636-80-6 | |
Description | CAS Common Chemistry is an open community resource for accessing chemical information. Nearly 500,000 chemical substances from CAS REGISTRY cover areas of community interest, including common and frequently regulated chemicals, and those relevant to high school and undergraduate chemistry classes. This chemical information, curated by our expert scientists, is provided in alignment with our mission as a division of the American Chemical Society. | |
Explanation | The data from CAS Common Chemistry is provided under a CC-BY-NC 4.0 license, unless otherwise stated. | |
Record name | 2-Pyridinecarboxylic acid, hydrochloride (1:1) | |
Source | ChemIDplus | |
URL | https://pubchem.ncbi.nlm.nih.gov/substance/?source=chemidplus&sourceid=0000636806 | |
Description | ChemIDplus is a free, web search system that provides access to the structure and nomenclature authority files used for the identification of chemical substances cited in National Library of Medicine (NLM) databases, including the TOXNET system. | |
Record name | 636-80-6 | |
Source | DTP/NCI | |
URL | https://dtp.cancer.gov/dtpstandard/servlet/dwindex?searchtype=NSC&outputformat=html&searchlist=363779 | |
Description | The NCI Development Therapeutics Program (DTP) provides services and resources to the academic and private-sector research communities worldwide to facilitate the discovery and development of new cancer therapeutic agents. | |
Explanation | Unless otherwise indicated, all text within NCI products is free of copyright and may be reused without our permission. Credit the National Cancer Institute as the source. | |
Record name | 2-Pyridinecarboxylic acid, hydrochloride (1:1) | |
Source | EPA Chemicals under the TSCA | |
URL | https://www.epa.gov/chemicals-under-tsca | |
Description | EPA Chemicals under the Toxic Substances Control Act (TSCA) collection contains information on chemicals and their regulations under TSCA, including non-confidential content from the TSCA Chemical Substance Inventory and Chemical Data Reporting. | |
Record name | Picolinic acid hydrochloride | |
Source | EPA DSSTox | |
URL | https://comptox.epa.gov/dashboard/DTXSID7060912 | |
Description | DSSTox provides a high quality public chemistry resource for supporting improved predictive toxicology. | |
Record name | Pyridine-2-carboxylic acid hydrochloride | |
Source | European Chemicals Agency (ECHA) | |
URL | https://echa.europa.eu/substance-information/-/substanceinfo/100.010.243 | |
Description | The European Chemicals Agency (ECHA) is an agency of the European Union which is the driving force among regulatory authorities in implementing the EU's groundbreaking chemicals legislation for the benefit of human health and the environment as well as for innovation and competitiveness. | |
Explanation | Use of the information, documents and data from the ECHA website is subject to the terms and conditions of this Legal Notice, and subject to other binding limitations provided for under applicable law, the information, documents and data made available on the ECHA website may be reproduced, distributed and/or used, totally or in part, for non-commercial purposes provided that ECHA is acknowledged as the source: "Source: European Chemicals Agency, http://echa.europa.eu/". Such acknowledgement must be included in each copy of the material. ECHA permits and encourages organisations and individuals to create links to the ECHA website under the following cumulative conditions: Links can only be made to webpages that provide a link to the Legal Notice page. | |
Record name | PICOLINIC ACID HYDROCHLORIDE | |
Source | FDA Global Substance Registration System (GSRS) | |
URL | https://gsrs.ncats.nih.gov/ginas/app/beta/substances/8HE8GCH2RD | |
Description | The FDA Global Substance Registration System (GSRS) enables the efficient and accurate exchange of information on what substances are in regulated products. Instead of relying on names, which vary across regulatory domains, countries, and regions, the GSRS knowledge base makes it possible for substances to be defined by standardized, scientific descriptions. | |
Explanation | Unless otherwise noted, the contents of the FDA website (www.fda.gov), both text and graphics, are not copyrighted. They are in the public domain and may be republished, reprinted and otherwise used freely by anyone without the need to obtain permission from FDA. Credit to the U.S. Food and Drug Administration as the source is appreciated but not required. | |
Retrosynthesis Analysis
AI-Powered Synthesis Planning: Our tool employs the Template_relevance Pistachio, Template_relevance Bkms_metabolic, Template_relevance Pistachio_ringbreaker, Template_relevance Reaxys, Template_relevance Reaxys_biocatalysis model, leveraging a vast database of chemical reactions to predict feasible synthetic routes.
One-Step Synthesis Focus: Specifically designed for one-step synthesis, it provides concise and direct routes for your target compounds, streamlining the synthesis process.
Accurate Predictions: Utilizing the extensive PISTACHIO, BKMS_METABOLIC, PISTACHIO_RINGBREAKER, REAXYS, REAXYS_BIOCATALYSIS database, our tool offers high-accuracy predictions, reflecting the latest in chemical research and data.
Strategy Settings
Precursor scoring | Relevance Heuristic |
---|---|
Min. plausibility | 0.01 |
Model | Template_relevance |
Template Set | Pistachio/Bkms_metabolic/Pistachio_ringbreaker/Reaxys/Reaxys_biocatalysis |
Top-N result to add to graph | 6 |
Feasible Synthetic Routes
Disclaimer and Information on In-Vitro Research Products
Please be aware that all articles and product information presented on BenchChem are intended solely for informational purposes. The products available for purchase on BenchChem are specifically designed for in-vitro studies, which are conducted outside of living organisms. In-vitro studies, derived from the Latin term "in glass," involve experiments performed in controlled laboratory settings using cells or tissues. It is important to note that these products are not categorized as medicines or drugs, and they have not received approval from the FDA for the prevention, treatment, or cure of any medical condition, ailment, or disease. We must emphasize that any form of bodily introduction of these products into humans or animals is strictly prohibited by law. It is essential to adhere to these guidelines to ensure compliance with legal and ethical standards in research and experimentation.