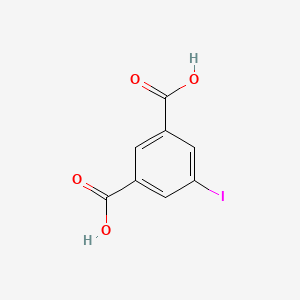
5-Iodoisophthalic acid
Overview
Description
5-Iodoisophthalic acid (C₈H₅IO₄, molecular weight 280.03 g/mol) is a derivative of isophthalic acid with an iodine atom substituted at the 5-position of the benzene ring (meta to both carboxylic acid groups). It is synthesized via hydrolysis of dimethyl 5-iodoisophthalate using lithium hydroxide and serves as a precursor for active esters (e.g., bis-N-hydroxysuccinimide esters) used in bioconjugation and radiopharmaceutical synthesis . The iodine atom enhances polarizability and facilitates applications in:
- Coordination chemistry: As a ligand in metallacrowns and metal-organic frameworks (MOFs) .
- Radiochemistry: As a tin precursor for radioiodination in imaging agents .
- Organic synthesis: For constructing π-conjugated systems and cyclophanes .
Its reactivity is defined by the electron-withdrawing iodine substituent, which lowers the pKa of the carboxylic acids (~2.5–3.0) compared to unsubstituted isophthalic acid (pKa₁ = 3.5, pKa₂ = 4.5) .
Biological Activity
5-Iodoisophthalic acid (5-IIPA) is a halogenated derivative of isophthalic acid, notable for its diverse biological activities and potential applications in medicinal chemistry. This article reviews the biological activity of 5-IIPA, drawing from various studies that highlight its interactions with biological systems, particularly in relation to ion transport, coordination chemistry, and potential therapeutic uses.
Chemical Structure and Properties
This compound is characterized by the presence of an iodine atom at the 5-position of the isophthalic acid structure. Its molecular formula is , and it features two carboxylic acid groups that can participate in various chemical reactions.
Property | Value |
---|---|
Molecular Weight | 286.03 g/mol |
Melting Point | 240-242 °C |
Solubility in Water | Low |
pKa | Approximately 4.5 |
Ion Transport Properties
Recent studies have demonstrated that derivatives of 5-IIPA, particularly in the form of halogen-bonded compounds, exhibit significant ion transport properties. A notable study focused on a 5-iodoisophthalamide system which showed proficient chloride-selective ion channel behavior within lipid bilayers. The di-hexyl-substituted derivative facilitated selective chloride transport via an antiport-mediated mechanism, emphasizing the role of halogen bonding in enhancing ion selectivity and transport efficiency .
Coordination Chemistry
This compound has been utilized to synthesize coordination polymers that exhibit unique structural and electronic properties. For instance, a study reported the formation of a two-dimensional Zn(II) coordination polymer based on 5-IIPA, revealing its potential applications in materials science and catalysis . These polymers are characterized by their layered structures and may possess interesting magnetic or electronic properties.
Anticancer Potential
The biological relevance of 5-IIPA extends to its potential anticancer properties. Research indicates that certain derivatives can interact with protein kinase C (PKC), a critical target in cancer therapy. In vitro studies have shown that these compounds can modify PKC activation, suggesting their utility as research tools or lead compounds for drug development aimed at cancer treatment .
Case Studies
- Ion Channel Activity : A study examining the ion transport capabilities of a 5-iodoisophthalamide derivative found that it formed a self-assembled structure conducive to chloride ion permeation across lipid bilayers. This was supported by molecular dynamics simulations which confirmed the significance of intermolecular hydrogen bonding within the channel .
- Coordination Polymers : The synthesis of coordination polymers from 5-IIPA has been explored, demonstrating how variations in ligand design can influence the properties of metal complexes formed with zinc or copper ions . These studies highlight the versatility of 5-IIPA in forming novel materials with potential applications in catalysis and sensing.
Scientific Research Applications
Coordination Chemistry
5-Iodoisophthalic acid serves as a ligand in the formation of metal-organic frameworks (MOFs) and coordination polymers. Its ability to coordinate with transition metals allows for the construction of complex structures with varied functionalities.
Case Study: Copper Coordination Polymers
A study reported the synthesis of seven copper coordination polymers utilizing this compound. These polymers exhibited distinct halogen-related bonding characteristics, which were modulated by the presence of nitrogen donor ligands . The structural analysis revealed that these complexes have potential applications in catalysis and materials science.
Data Table: Properties of Copper Coordination Polymers
Polymer Type | Metal | Ligand | Notable Property |
---|---|---|---|
Polymer 1 | Cu | This compound | Halogen bonding interactions |
Polymer 2 | Cu | Nitrogen donor ligands | Enhanced stability |
Ion Transport Mechanisms
Recent research has highlighted the role of 5-iodoisophthalic derivatives in developing artificial ion channels. These channels are crucial for mimicking biological ion transport mechanisms.
Case Study: Chloride-Selective Channels
A study demonstrated that a di-hexyl-substituted derivative of 5-iodoisophthalamide exhibited significant chloride transport across lipid bilayer membranes. The mechanism was identified as antiport-mediated, facilitated by halogen bonding and hydrogen bonding within the channel structure . This finding underscores the potential for developing selective ion transport systems based on halogenated compounds.
Material Science Applications
The incorporation of this compound into polymer matrices has been explored for its impact on material properties.
Case Study: Two-Dimensional ZnII Coordination Polymer
Research involving zinc(II) coordination with this compound led to the formation of a layered coordination polymer. This material demonstrated unique electronic properties, making it suitable for applications in electronic devices and sensors .
Data Table: Properties of ZnII Coordination Polymer
Property | Value |
---|---|
Layer Thickness | X nanometers |
Conductivity | Y S/cm |
Band Gap | Z eV |
Biochemical Applications
The biochemical implications of this compound derivatives are also noteworthy, particularly in drug design and development.
Case Study: Hydrogen Bonded Systems
Investigations into hydrogen-bonded systems involving this compound revealed insights into molecular interactions that can be leveraged for pharmaceutical applications. The ability to form stable complexes with biological targets positions this compound as a candidate for drug development .
Chemical Reactions Analysis
Types of Chemical Reactions
2-Amino-5-iodoisophthalic acid can participate in several types of chemical reactions due to the presence of the amino and iodine substituents.
Oxidation Reactions
-
The amino group can be converted into a nitro group or other oxidized forms.
-
Common oxidizing agents include potassium permanganate () and hydrogen peroxide ().
Reduction Reactions
-
The compound can be reduced to form derivatives with different functional groups.
-
Reducing agents such as sodium borohydride () or lithium aluminum hydride () are typically used.
Substitution Reactions
-
The iodine atom can be substituted with other groups through nucleophilic substitution reactions.
-
Nucleophiles such as thiols, amines, or alkoxides can be used.
Reactions towards 5-amino-2,4,6-triiodoisophthaloyl dichloride
5-amino-2,4,6-triiodoisophthaloyl dichloride, a derivative, is synthesized using m-phthalic acid as a starting material with a chloride reagent and an initiator with solid phosgene .
Reaction: Acyl chloride reaction with 5-amino-2,4,6-triiodoisophthalic acids to prepare 5-amino-2,4,6-triiodoisophthalic acid acyl chlorides .
Reagents :
Uses:
Q & A
Basic Research Questions
Q. What are the standard synthetic routes for 5-iodoisophthalic acid, and how can its purity be validated?
The compound is typically synthesized via hydrolysis of dimethyl 5-iodoisophthalate using lithium hydroxide (LiOH) in a methanol/water mixture, followed by purification via recrystallization . For purity validation, combine techniques such as:
- HPLC : Monitor for hydrolysis byproducts (e.g., residual esters).
- Mass spectrometry (MS) : Confirm molecular weight (MW 292.01 g/mol).
- Elemental analysis : Verify iodine content (~43.5% theoretical). Basic characterization should reference known spectral data (e.g., IR carbonyl peaks at ~1680–1700 cm⁻¹) .
Q. What safety precautions are critical when handling this compound in laboratory settings?
- Use personal protective equipment (PPE) : Nitrile gloves, lab coats, and safety goggles.
- Work in a fume hood to avoid inhalation of fine particles.
- Store in airtight containers under inert gas (e.g., argon) to prevent hydrolysis or oxidation .
Q. Which spectroscopic methods are most reliable for structural confirmation of this compound derivatives?
- 1H NMR : Identify aromatic proton environments (e.g., meta-substitution patterns).
- 13C NMR : Confirm carboxylic acid carbons (~170 ppm) and iodinated aromatic carbons. Note: Peak broadening in NMR due to iodine’s quadrupolar moment may complicate interpretation; compare with computational models (DFT) for ambiguous cases .
Advanced Research Questions
Q. How can hydrolysis susceptibility of this compound derivatives during HPLC analysis be mitigated?
- Optimize mobile phase : Use acidic conditions (e.g., 0.1% trifluoroacetic acid in acetonitrile/water) to stabilize ester groups.
- Lower column temperature : Reduce hydrolysis kinetics (e.g., 15–20°C).
- Shorten run times : Minimize exposure to aqueous environments .
Q. What strategies resolve contradictions in reported NMR data for DOTA-functionalized derivatives of this compound?
- Cross-validate spectra : Compare data across multiple solvents (DMSO-d6 vs. CDCl3) to assess solvent-induced shifts.
- Supplement with HRMS : Confirm molecular formulas when peak overlaps occur (e.g., aromatic vs. aliphatic regions).
- Reference synthetic protocols : Ensure consistent protection/deprotection steps (e.g., t-butyl vs. benzyl esters) .
Q. How can AI-driven retrosynthesis tools improve the design of novel this compound-based conjugates?
Platforms like Pistachio or Reaxys leverage reaction databases to propose:
- Functionalization sites : Prioritize iodination at the 5-position for steric accessibility.
- Spacer integration : Use hexanediamine linkers for DOTA chelator attachment (see Scheme 3 in ).
- Stability screening : Predict hydrolytic degradation risks using QSPR models .
Q. What experimental controls are essential when comparing catalytic iodination methods for isophthalic acid derivatives?
- Blank reactions : Exclude catalysts to assess inherent iodination rates.
- Isotope tracing : Use ¹²⁵I-labeled reagents to track incorporation efficiency.
- Side-product analysis : Monitor for di-iodinated byproducts via LC-MS .
Q. Methodological Considerations
Comparison with Similar Compounds
Comparison with Structurally Similar Compounds
Table 1: Structural and Functional Comparison
Key Comparisons
Electronic Effects: The iodine atom in this compound increases electron density withdrawal compared to methyl or amino groups, enhancing acidity and reactivity in nucleophilic substitutions . Fluorine’s higher electronegativity (vs. iodine) reduces steric bulk but limits polarizability, making 5-fluoroisophthalic acid less suited for radiochemistry but useful in hydrogen-bonded networks .
Synthetic Utility: this compound’s iodine enables Stille couplings and radioiodination via tin precursors , whereas 5-cyanophenyl derivatives participate in π-stacking for MOF stabilization . Triiodinated derivatives (e.g., 5-amino-2,4,6-triiodoisophthalic acid) are bulkier, favoring non-covalent interactions in contrast agents .
Material Applications :
Properties
IUPAC Name |
5-iodobenzene-1,3-dicarboxylic acid | |
---|---|---|
Source | PubChem | |
URL | https://pubchem.ncbi.nlm.nih.gov | |
Description | Data deposited in or computed by PubChem | |
InChI |
InChI=1S/C8H5IO4/c9-6-2-4(7(10)11)1-5(3-6)8(12)13/h1-3H,(H,10,11)(H,12,13) | |
Source | PubChem | |
URL | https://pubchem.ncbi.nlm.nih.gov | |
Description | Data deposited in or computed by PubChem | |
InChI Key |
QMDFYAWUWIIQFM-UHFFFAOYSA-N | |
Source | PubChem | |
URL | https://pubchem.ncbi.nlm.nih.gov | |
Description | Data deposited in or computed by PubChem | |
Canonical SMILES |
C1=C(C=C(C=C1C(=O)O)I)C(=O)O | |
Source | PubChem | |
URL | https://pubchem.ncbi.nlm.nih.gov | |
Description | Data deposited in or computed by PubChem | |
Molecular Formula |
C8H5IO4 | |
Source | PubChem | |
URL | https://pubchem.ncbi.nlm.nih.gov | |
Description | Data deposited in or computed by PubChem | |
DSSTOX Substance ID |
DTXSID3068675 | |
Record name | 5-Iodoisophthalic acid | |
Source | EPA DSSTox | |
URL | https://comptox.epa.gov/dashboard/DTXSID3068675 | |
Description | DSSTox provides a high quality public chemistry resource for supporting improved predictive toxicology. | |
Molecular Weight |
292.03 g/mol | |
Source | PubChem | |
URL | https://pubchem.ncbi.nlm.nih.gov | |
Description | Data deposited in or computed by PubChem | |
CAS No. |
51839-16-8 | |
Record name | 5-Iodo-1,3-benzenedicarboxylic acid | |
Source | CAS Common Chemistry | |
URL | https://commonchemistry.cas.org/detail?cas_rn=51839-16-8 | |
Description | CAS Common Chemistry is an open community resource for accessing chemical information. Nearly 500,000 chemical substances from CAS REGISTRY cover areas of community interest, including common and frequently regulated chemicals, and those relevant to high school and undergraduate chemistry classes. This chemical information, curated by our expert scientists, is provided in alignment with our mission as a division of the American Chemical Society. | |
Explanation | The data from CAS Common Chemistry is provided under a CC-BY-NC 4.0 license, unless otherwise stated. | |
Record name | 1,3-Benzenedicarboxylic acid, 5-iodo- | |
Source | ChemIDplus | |
URL | https://pubchem.ncbi.nlm.nih.gov/substance/?source=chemidplus&sourceid=0051839168 | |
Description | ChemIDplus is a free, web search system that provides access to the structure and nomenclature authority files used for the identification of chemical substances cited in National Library of Medicine (NLM) databases, including the TOXNET system. | |
Record name | 1,3-Benzenedicarboxylic acid, 5-iodo- | |
Source | EPA Chemicals under the TSCA | |
URL | https://www.epa.gov/chemicals-under-tsca | |
Description | EPA Chemicals under the Toxic Substances Control Act (TSCA) collection contains information on chemicals and their regulations under TSCA, including non-confidential content from the TSCA Chemical Substance Inventory and Chemical Data Reporting. | |
Record name | 5-Iodoisophthalic acid | |
Source | EPA DSSTox | |
URL | https://comptox.epa.gov/dashboard/DTXSID3068675 | |
Description | DSSTox provides a high quality public chemistry resource for supporting improved predictive toxicology. | |
Record name | 5-iodoisophthalic acid | |
Source | European Chemicals Agency (ECHA) | |
URL | https://echa.europa.eu/substance-information/-/substanceinfo/100.052.225 | |
Description | The European Chemicals Agency (ECHA) is an agency of the European Union which is the driving force among regulatory authorities in implementing the EU's groundbreaking chemicals legislation for the benefit of human health and the environment as well as for innovation and competitiveness. | |
Explanation | Use of the information, documents and data from the ECHA website is subject to the terms and conditions of this Legal Notice, and subject to other binding limitations provided for under applicable law, the information, documents and data made available on the ECHA website may be reproduced, distributed and/or used, totally or in part, for non-commercial purposes provided that ECHA is acknowledged as the source: "Source: European Chemicals Agency, http://echa.europa.eu/". Such acknowledgement must be included in each copy of the material. ECHA permits and encourages organisations and individuals to create links to the ECHA website under the following cumulative conditions: Links can only be made to webpages that provide a link to the Legal Notice page. | |
Retrosynthesis Analysis
AI-Powered Synthesis Planning: Our tool employs the Template_relevance Pistachio, Template_relevance Bkms_metabolic, Template_relevance Pistachio_ringbreaker, Template_relevance Reaxys, Template_relevance Reaxys_biocatalysis model, leveraging a vast database of chemical reactions to predict feasible synthetic routes.
One-Step Synthesis Focus: Specifically designed for one-step synthesis, it provides concise and direct routes for your target compounds, streamlining the synthesis process.
Accurate Predictions: Utilizing the extensive PISTACHIO, BKMS_METABOLIC, PISTACHIO_RINGBREAKER, REAXYS, REAXYS_BIOCATALYSIS database, our tool offers high-accuracy predictions, reflecting the latest in chemical research and data.
Strategy Settings
Precursor scoring | Relevance Heuristic |
---|---|
Min. plausibility | 0.01 |
Model | Template_relevance |
Template Set | Pistachio/Bkms_metabolic/Pistachio_ringbreaker/Reaxys/Reaxys_biocatalysis |
Top-N result to add to graph | 6 |
Feasible Synthetic Routes
Disclaimer and Information on In-Vitro Research Products
Please be aware that all articles and product information presented on BenchChem are intended solely for informational purposes. The products available for purchase on BenchChem are specifically designed for in-vitro studies, which are conducted outside of living organisms. In-vitro studies, derived from the Latin term "in glass," involve experiments performed in controlled laboratory settings using cells or tissues. It is important to note that these products are not categorized as medicines or drugs, and they have not received approval from the FDA for the prevention, treatment, or cure of any medical condition, ailment, or disease. We must emphasize that any form of bodily introduction of these products into humans or animals is strictly prohibited by law. It is essential to adhere to these guidelines to ensure compliance with legal and ethical standards in research and experimentation.