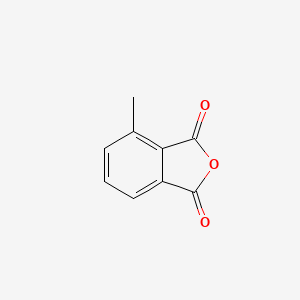
3-Methylphthalic anhydride
Overview
Description
3-Methylphthalic anhydride, also known as 4-methyl-2-benzofuran-1,3-dione, is an organic compound with the molecular formula C9H6O3. It is a derivative of phthalic anhydride, where a methyl group is substituted at the third position of the aromatic ring. This compound is typically found in the form of white to pale yellow crystals or powder and is known for its reactivity and utility in various chemical processes .
Mechanism of Action
Target of Action
3-Methylphthalic anhydride is a chemical compound that primarily targets organic compounds in chemical reactions . It is known to interact with compounds such as Methylamine hydrochloride .
Mode of Action
The mode of action of this compound involves a nucleophilic attack on the carbonyl group, followed by the removal of a leaving group . This is a common mechanism for acid anhydrides, which are a group of carboxylic acid derivatives .
Biochemical Pathways
This compound can react with Methylamine hydrochloride to produce 2,3-Dihydro-2,4-dimethyl-1H-isoindole-1,3-dione . This reaction is part of the broader biochemical pathways involving carboxylic acid derivatives .
Pharmacokinetics
It is known that the compound is moisture-sensitive and reacts with water , which could influence its bioavailability.
Result of Action
The result of the action of this compound is the formation of 2,3-Dihydro-2,4-dimethyl-1H-isoindole-1,3-dione when it reacts with Methylamine hydrochloride . This product may have various applications in chemical and pharmaceutical industries.
Action Environment
The action of this compound is influenced by environmental factors such as moisture and temperature . The compound is moisture-sensitive and should be stored under dry inert gas . Its reactivity with water suggests that its action, efficacy, and stability could be significantly affected in humid environments .
Biochemical Analysis
Biochemical Properties
It is known that this compound can react with Methylamine hydrochloride to produce 2,3-Dihydro-2,4-dimethyl-1H-isoindole-1,3-dione . The exact enzymes, proteins, and other biomolecules it interacts with are yet to be identified.
Cellular Effects
3-Methylphthalic anhydride has been shown to have beneficial effects when compared to petrochemical phthalic anhydride in end-user products such as coatings, pigments, polyurethanes, and lubricants . It has also been suggested to have anticancer activity and can inhibit cancer cell growth by interfering with the synthesis of DNA and RNA
Metabolic Pathways
It is known that phthalates can be biodegraded, and this process involves various enzymes . The specific enzymes or cofactors that this compound interacts with, as well as any effects on metabolic flux or metabolite levels, are yet to be identified.
Preparation Methods
Synthetic Routes and Reaction Conditions: The synthesis of 3-Methylphthalic anhydride can be achieved through several methods. One common approach involves the oxidation of 3-methylphthalic acid using dehydrating agents such as acetic anhydride or sulfuric acid. Another method includes the catalytic oxidation of 3-methyl-o-xylene in the presence of a vanadium pentoxide catalyst .
Industrial Production Methods: In industrial settings, this compound is often produced via the catalytic oxidation of 3-methyl-o-xylene. This process involves the use of air or oxygen in the presence of a catalyst, typically vanadium pentoxide, at elevated temperatures. The resulting product is then purified through distillation or recrystallization .
Chemical Reactions Analysis
Types of Reactions: 3-Methylphthalic anhydride undergoes various chemical reactions, including:
Hydrolysis: Reacts with water to form 3-methylphthalic acid.
Aminolysis: Reacts with amines to form amides.
Alcoholysis: Reacts with alcohols to form esters.
Common Reagents and Conditions:
Hydrolysis: Typically carried out in the presence of water or aqueous solutions.
Aminolysis: Conducted using primary or secondary amines under mild heating.
Alcoholysis: Performed with alcohols such as methanol or ethanol, often in the presence of an acid catalyst.
Major Products Formed:
Hydrolysis: 3-Methylphthalic acid.
Aminolysis: 3-Methylphthalimide.
Alcoholysis: 3-Methylphthalate esters.
Scientific Research Applications
3-Methylphthalic anhydride has a wide range of applications in scientific research and industry:
Chemistry: Used as an intermediate in the synthesis of various organic compounds, including dyes, pigments, and pharmaceuticals.
Biology: Employed in the preparation of biologically active molecules and as a reagent in biochemical assays.
Medicine: Utilized in the synthesis of drug molecules and as a building block for medicinal chemistry.
Industry: Applied in the production of plasticizers, resins, and coatings
Comparison with Similar Compounds
Phthalic anhydride: The parent compound without the methyl substitution.
4-Methylphthalic anhydride: A positional isomer with the methyl group at the fourth position.
2,3-Toluenedicarboxylic anhydride: Another derivative with two carboxylic anhydride groups
Uniqueness: 3-Methylphthalic anhydride is unique due to its specific substitution pattern, which imparts distinct reactivity and properties compared to its analogs. The presence of the methyl group at the third position influences its chemical behavior, making it a valuable intermediate in various synthetic applications .
Properties
IUPAC Name |
4-methyl-2-benzofuran-1,3-dione | |
---|---|---|
Source | PubChem | |
URL | https://pubchem.ncbi.nlm.nih.gov | |
Description | Data deposited in or computed by PubChem | |
InChI |
InChI=1S/C9H6O3/c1-5-3-2-4-6-7(5)9(11)12-8(6)10/h2-4H,1H3 | |
Source | PubChem | |
URL | https://pubchem.ncbi.nlm.nih.gov | |
Description | Data deposited in or computed by PubChem | |
InChI Key |
TWWAWPHAOPTQEU-UHFFFAOYSA-N | |
Source | PubChem | |
URL | https://pubchem.ncbi.nlm.nih.gov | |
Description | Data deposited in or computed by PubChem | |
Canonical SMILES |
CC1=C2C(=CC=C1)C(=O)OC2=O | |
Source | PubChem | |
URL | https://pubchem.ncbi.nlm.nih.gov | |
Description | Data deposited in or computed by PubChem | |
Molecular Formula |
C9H6O3 | |
Source | PubChem | |
URL | https://pubchem.ncbi.nlm.nih.gov | |
Description | Data deposited in or computed by PubChem | |
DSSTOX Substance ID |
DTXSID0063606 | |
Record name | 1,3-Isobenzofurandione, 4-methyl- | |
Source | EPA DSSTox | |
URL | https://comptox.epa.gov/dashboard/DTXSID0063606 | |
Description | DSSTox provides a high quality public chemistry resource for supporting improved predictive toxicology. | |
Molecular Weight |
162.14 g/mol | |
Source | PubChem | |
URL | https://pubchem.ncbi.nlm.nih.gov | |
Description | Data deposited in or computed by PubChem | |
CAS No. |
4792-30-7, 30140-42-2 | |
Record name | 3-Methylphthalic anhydride | |
Source | CAS Common Chemistry | |
URL | https://commonchemistry.cas.org/detail?cas_rn=4792-30-7 | |
Description | CAS Common Chemistry is an open community resource for accessing chemical information. Nearly 500,000 chemical substances from CAS REGISTRY cover areas of community interest, including common and frequently regulated chemicals, and those relevant to high school and undergraduate chemistry classes. This chemical information, curated by our expert scientists, is provided in alignment with our mission as a division of the American Chemical Society. | |
Explanation | The data from CAS Common Chemistry is provided under a CC-BY-NC 4.0 license, unless otherwise stated. | |
Record name | 4-Methyl-1,3-isobenzofurandione | |
Source | ChemIDplus | |
URL | https://pubchem.ncbi.nlm.nih.gov/substance/?source=chemidplus&sourceid=0004792307 | |
Description | ChemIDplus is a free, web search system that provides access to the structure and nomenclature authority files used for the identification of chemical substances cited in National Library of Medicine (NLM) databases, including the TOXNET system. | |
Record name | 1,3-Isobenzofurandione, methyl- | |
Source | ChemIDplus | |
URL | https://pubchem.ncbi.nlm.nih.gov/substance/?source=chemidplus&sourceid=0030140422 | |
Description | ChemIDplus is a free, web search system that provides access to the structure and nomenclature authority files used for the identification of chemical substances cited in National Library of Medicine (NLM) databases, including the TOXNET system. | |
Record name | 3-Methylphthalic anhydride | |
Source | DTP/NCI | |
URL | https://dtp.cancer.gov/dtpstandard/servlet/dwindex?searchtype=NSC&outputformat=html&searchlist=61875 | |
Description | The NCI Development Therapeutics Program (DTP) provides services and resources to the academic and private-sector research communities worldwide to facilitate the discovery and development of new cancer therapeutic agents. | |
Explanation | Unless otherwise indicated, all text within NCI products is free of copyright and may be reused without our permission. Credit the National Cancer Institute as the source. | |
Record name | 1,3-Isobenzofurandione, 4-methyl- | |
Source | EPA Chemicals under the TSCA | |
URL | https://www.epa.gov/chemicals-under-tsca | |
Description | EPA Chemicals under the Toxic Substances Control Act (TSCA) collection contains information on chemicals and their regulations under TSCA, including non-confidential content from the TSCA Chemical Substance Inventory and Chemical Data Reporting. | |
Record name | 1,3-Isobenzofurandione, 4-methyl- | |
Source | EPA DSSTox | |
URL | https://comptox.epa.gov/dashboard/DTXSID0063606 | |
Description | DSSTox provides a high quality public chemistry resource for supporting improved predictive toxicology. | |
Record name | 3-methylphthalic anhydride | |
Source | European Chemicals Agency (ECHA) | |
URL | https://echa.europa.eu/substance-information/-/substanceinfo/100.023.040 | |
Description | The European Chemicals Agency (ECHA) is an agency of the European Union which is the driving force among regulatory authorities in implementing the EU's groundbreaking chemicals legislation for the benefit of human health and the environment as well as for innovation and competitiveness. | |
Explanation | Use of the information, documents and data from the ECHA website is subject to the terms and conditions of this Legal Notice, and subject to other binding limitations provided for under applicable law, the information, documents and data made available on the ECHA website may be reproduced, distributed and/or used, totally or in part, for non-commercial purposes provided that ECHA is acknowledged as the source: "Source: European Chemicals Agency, http://echa.europa.eu/". Such acknowledgement must be included in each copy of the material. ECHA permits and encourages organisations and individuals to create links to the ECHA website under the following cumulative conditions: Links can only be made to webpages that provide a link to the Legal Notice page. | |
Record name | 4-METHYL-1,3-ISOBENZOFURANDIONE | |
Source | FDA Global Substance Registration System (GSRS) | |
URL | https://gsrs.ncats.nih.gov/ginas/app/beta/substances/BJC50EL71I | |
Description | The FDA Global Substance Registration System (GSRS) enables the efficient and accurate exchange of information on what substances are in regulated products. Instead of relying on names, which vary across regulatory domains, countries, and regions, the GSRS knowledge base makes it possible for substances to be defined by standardized, scientific descriptions. | |
Explanation | Unless otherwise noted, the contents of the FDA website (www.fda.gov), both text and graphics, are not copyrighted. They are in the public domain and may be republished, reprinted and otherwise used freely by anyone without the need to obtain permission from FDA. Credit to the U.S. Food and Drug Administration as the source is appreciated but not required. | |
Synthesis routes and methods
Procedure details
Retrosynthesis Analysis
AI-Powered Synthesis Planning: Our tool employs the Template_relevance Pistachio, Template_relevance Bkms_metabolic, Template_relevance Pistachio_ringbreaker, Template_relevance Reaxys, Template_relevance Reaxys_biocatalysis model, leveraging a vast database of chemical reactions to predict feasible synthetic routes.
One-Step Synthesis Focus: Specifically designed for one-step synthesis, it provides concise and direct routes for your target compounds, streamlining the synthesis process.
Accurate Predictions: Utilizing the extensive PISTACHIO, BKMS_METABOLIC, PISTACHIO_RINGBREAKER, REAXYS, REAXYS_BIOCATALYSIS database, our tool offers high-accuracy predictions, reflecting the latest in chemical research and data.
Strategy Settings
Precursor scoring | Relevance Heuristic |
---|---|
Min. plausibility | 0.01 |
Model | Template_relevance |
Template Set | Pistachio/Bkms_metabolic/Pistachio_ringbreaker/Reaxys/Reaxys_biocatalysis |
Top-N result to add to graph | 6 |
Feasible Synthetic Routes
Q1: What are the typical applications of 3-methylphthalic anhydride?
A1: this compound is a key building block for synthesizing various compounds. One prominent application is in producing diesters, which can serve as alternative plasticizers []. Additionally, it acts as a precursor in synthesizing derivatives of kermesic and isokermesic acids, natural dyes found in insects [].
Q2: How is the kinetics of this compound's reactions typically studied?
A2: Researchers successfully employed Attenuated Total Reflection Fourier Transformed Infrared Spectroscopy (ATR-FTIR) as an inline analytical technique to investigate the kinetics of this compound esterification with 2-ethylhexanol []. This method, coupled with chemometric modeling like Indirect Hard Modeling (IHM), allows for real-time monitoring and characterization of the reaction progression.
Q3: Can you describe a specific reaction involving this compound and its kinetic parameters?
A3: The acid-catalyzed esterification of this compound with 2-ethylhexanol is a two-step process. The second esterification step, considered the rate-limiting step, exhibits pseudo-first-order kinetics concerning monoester formation. The activation energy for this step was determined to be 79.5 kJ mol-1 [].
Q4: How does the structure of this compound influence its reactivity?
A4: The molecule of this compound exhibits a non-planar structure, with a slight dihedral angle between the benzene ring and the fused five-membered heterocyclic ring. This structural feature, along with distortions in the benzene ring bond angles caused by the heterocyclic ring, influences the compound's reactivity [].
Q5: Are there alternative synthetic routes to this compound?
A5: Yes, an improved synthesis method involves the Diels-Alder reaction of 2-methylfuran and maleic anhydride. Subsequent hydrogenation and tandem catalytic aromatization using a combination of zeolite H-Y and Pd/C yield this compound, alongside other aromatic byproducts like o- and m-toluic acid [].
Q6: How does the choice of catalyst system influence product selectivity in the synthesis of this compound?
A6: Research shows that the acidity and textural properties of the solid acid catalyst significantly impact product selectivity during the aromatization of the hydrogenated Diels-Alder adduct of 2-methylfuran and maleic anhydride []. For instance, a balanced catalyst acidity and porosity, achievable by tuning the ratio of strong to total acid sites and the mesopore volume in zeolite H-Y, favors this compound formation.
Q7: Can the decarboxylation byproduct formation be minimized during this compound synthesis?
A7: Yes, optimizing the catalyst system can effectively suppress decarboxylation. For example, strategically adjusting the zeolite-to-Pd/C ratio enables efficient coupling of dehydration and dehydrogenation steps, leading to a higher yield of this compound relative to total aromatic products [].
Q8: How can the dielectric properties of polymers derived from this compound be studied?
A8: Impedance measurements provide valuable insights into the dielectric properties and curing kinetics of epoxy resins, including those formed using this compound as a curing agent. Researchers have employed this method, using a thin layer of the curing resin between capacitor plates, to characterize the evolving dielectric behavior of the material during the curing process [].
Q9: Are there alternative uses for this compound in polymer chemistry beyond epoxy resins?
A9: this compound serves as a starting material for synthesizing oligomers containing luminol-like structures. These oligomers, while exhibiting significantly lower chemiluminescence efficiency than luminol itself, offer insights into the impact of chromophore proximity on luminescence properties [].
Disclaimer and Information on In-Vitro Research Products
Please be aware that all articles and product information presented on BenchChem are intended solely for informational purposes. The products available for purchase on BenchChem are specifically designed for in-vitro studies, which are conducted outside of living organisms. In-vitro studies, derived from the Latin term "in glass," involve experiments performed in controlled laboratory settings using cells or tissues. It is important to note that these products are not categorized as medicines or drugs, and they have not received approval from the FDA for the prevention, treatment, or cure of any medical condition, ailment, or disease. We must emphasize that any form of bodily introduction of these products into humans or animals is strictly prohibited by law. It is essential to adhere to these guidelines to ensure compliance with legal and ethical standards in research and experimentation.