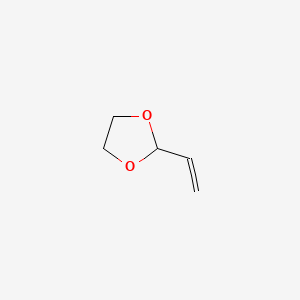
2-Vinyl-1,3-dioxolane
Overview
Description
It is a colorless liquid with a boiling point of 115-116°C and a density of 1.001 g/mL at 25°C . This compound is characterized by the presence of a vinyl group attached to a 1,3-dioxolane ring, making it a versatile intermediate in organic synthesis.
Preparation Methods
Synthetic Routes and Reaction Conditions: 2-Vinyl-1,3-dioxolane can be synthesized through the radical vinylation of 1,3-dioxolanes using vinyl bromides. This reaction typically involves the use of vinyl bromides with an electron-withdrawing substituent at the β-position, proceeding smoothly to give 2-vinyl-1,3-dioxolanes in good yields . Another method involves the reaction of acrolein with ethylene glycol in the presence of a solid acid catalyst at temperatures below 50°C .
Industrial Production Methods: Industrial production of this compound follows similar synthetic routes but on a larger scale. The reaction mixture, typically containing acrolein, ethylene glycol, and the desired product, is continuously circulated at controlled temperatures to optimize yield and purity .
Chemical Reactions Analysis
Types of Reactions: 2-Vinyl-1,3-dioxolane undergoes various chemical reactions, including:
Oxidation: It can be oxidized using agents like potassium permanganate (KMnO4) or osmium tetroxide (OsO4).
Reduction: Reduction can be achieved using hydrogen gas in the presence of nickel or rhodium catalysts.
Common Reagents and Conditions:
Oxidation: KMnO4, OsO4
Reduction: H2/Ni, H2/Rh
Substitution: LiAlH4, NaBH4
Major Products: The major products formed from these reactions depend on the specific conditions and reagents used. For example, oxidation may yield carboxylic acids, while reduction typically produces alcohols .
Scientific Research Applications
Synthesis and Reactivity
The synthesis of 2-vinyl-1,3-dioxolane typically involves the vinylation of readily available 1,3-dioxolanes using vinyl bromides. A notable study demonstrated that radical vinylation could produce this compound efficiently under optimized conditions. The reaction mechanism involves:
- Formation of a radical from a radical initiator.
- Addition of the resultant radical to the vinyl bromide.
- Elimination to yield the vinylated product.
The yields of this reaction can vary based on the substituents on the vinyl bromide and the structure of the dioxolane used .
Vinylation Reactions
This compound serves as an important intermediate in organic synthesis. It can be further transformed into various functionalized compounds through:
- Hydrolysis : Converting it into unsaturated carbonyl compounds.
- Cross-coupling reactions : Serving as a vinyl donor in reactions with electrophiles.
Polymer Chemistry
Due to its vinyl group, this compound can participate in polymerization reactions, leading to the formation of polymers with tailored properties. Its use in copolymerization with other monomers can enhance material characteristics such as thermal stability and mechanical strength.
Pharmaceutical Applications
The compound's structure allows for modifications that can lead to biologically active molecules. Research has shown potential applications in drug design where the dioxolane moiety contributes to the pharmacological profile of new compounds.
Case Study 1: Radical Vinylation
In a study by Kippo et al., the radical vinylation of various dioxolanes was investigated using vinyl bromides as reactants. The results indicated that:
- The reaction conditions significantly influenced yield.
- Dioxolanes with different alkyl groups showed varying reactivity, demonstrating the importance of substituent effects on radical stability and reactivity .
Dioxolane Structure | Vinyl Bromide Used | Yield (%) |
---|---|---|
2-Octyl | Methyl 3-bromo-2-methylacrylate | 68 |
Cyclohexyl | Methyl 3-bromo-2-methylacrylate | 56 |
2-Aryl | Methyl 3-bromoacrylate | 85 |
Case Study 2: Polymerization
Research has shown that when incorporated into polymeric structures, this compound enhances properties such as flexibility and resistance to thermal degradation. This has implications for developing new materials for industrial applications.
Mechanism of Action
The mechanism of action of 2-vinyl-1,3-dioxolane involves its ability to undergo polymerization and other chemical transformations. The vinyl group allows it to participate in radical and ionic polymerization reactions, forming polymers with diverse properties . The 1,3-dioxolane ring provides stability and reactivity, enabling its use in various synthetic applications.
Comparison with Similar Compounds
- 4-Vinyl-1,3-dioxolan-2-one
- 4,4,6-Trimethyl-2-vinyl-1,3-dioxane
- Vinylene carbonate
Comparison: 2-Vinyl-1,3-dioxolane is unique due to its specific structure, which combines a vinyl group with a 1,3-dioxolane ring. This combination imparts distinct reactivity and stability compared to similar compounds. For instance, 4-vinyl-1,3-dioxolan-2-one has a different ring structure, affecting its polymerization behavior and applications .
Biological Activity
2-Vinyl-1,3-dioxolane is an organic compound belonging to the dioxolane family, characterized by a five-membered ring containing two oxygen atoms and a vinyl group. This compound has garnered attention due to its potential biological activities, particularly in the fields of antimicrobial, anticancer, and cytotoxic applications. This article synthesizes current research findings on the biological activity of this compound, including data tables and case studies.
Synthesis of this compound
The synthesis of this compound typically involves the vinylation of 1,3-dioxolanes using vinyl bromides under radical conditions. For instance, one study optimized the reaction conditions to achieve yields of up to 68% for the vinylated product using di-tert-butylhyponitrite as a radical initiator .
Antimicrobial Activity
Research indicates that derivatives of 1,3-dioxolanes exhibit significant antibacterial and antifungal properties. A study assessed various synthesized dioxolane compounds for their in vitro activity against several bacterial strains and fungi. The results showed that compounds similar to this compound demonstrated effective antibacterial activity against Gram-positive bacteria such as Staphylococcus aureus and Enterococcus faecalis, with minimum inhibitory concentration (MIC) values ranging from 625 to 1250 µg/mL .
Table 1: Antimicrobial Activity of Dioxolane Derivatives
Compound | Bacteria Tested | MIC (µg/mL) |
---|---|---|
This compound | S. aureus | 625-1250 |
S. epidermidis | 500-1000 | |
E. faecalis | 750 | |
P. aeruginosa | 1000 | |
C. albicans (fungi) | 250 |
Anticancer Activity
The compound has also been evaluated for its cytotoxic effects against various cancer cell lines. A notable study highlighted that poly(maleic anhydride-alt-2-vinyl-1,3-dioxolane) exhibited significant cytotoxicity against colorectal adenocarcinoma cells (Caco-2), indicating its potential as an anticancer agent .
Case Study: Cytotoxicity Against Cancer Cell Lines
In vitro studies showed that the dioxolane derivatives could modulate multidrug resistance in tumors by interacting with P-glycoprotein, a key factor in drug resistance . The most effective compounds were those with specific structural modifications that enhanced their interaction with cancer cells.
Structure-Activity Relationship
The structure-activity relationship (SAR) of dioxolanes indicates that modifications in the dioxolane ring can significantly influence biological activity. For example, the introduction of different substituents on the dioxolane ring has been associated with enhanced antimicrobial and anticancer properties .
Table 2: Structure-Activity Relationship of Dioxolanes
Modification | Biological Activity | Effectiveness |
---|---|---|
Alkyl substitution | Increased antibacterial activity | High |
Aromatic substitution | Enhanced cytotoxicity against cancer cells | Moderate |
Halogenation | Improved antifungal properties | High |
Q & A
Basic Research Questions
Q. What are the key physicochemical properties of 2-vinyl-1,3-dioxolane, and how do they influence experimental design?
- Answer : The compound's boiling point (115–116°C), density (1.001 g/cm³), and flash point (14°C) dictate handling protocols. For example, its low flash point necessitates inert atmospheres (e.g., nitrogen) during reactions to prevent flammability. The moderate boiling point allows distillation as a purification method, while its density informs solvent layering in biphasic systems. Refractive index (1.430) can aid in purity assessment via refractometry.
Q. What synthetic routes are commonly used to prepare this compound, and what are their advantages?
- Answer : A standard method involves acid-catalyzed acetal formation between acrolein and ethylene glycol. For example, p-toluenesulfonic acid catalyzes the reaction, yielding this compound with high regioselectivity . This route is scalable and avoids harsh conditions, making it suitable for lab-scale synthesis. Alternative glycols (e.g., substituted diols) can modify the dioxolane ring for tailored applications .
Advanced Research Questions
Q. How does the structural rigidity of this compound influence its reactivity in radical or cationic polymerization?
- Answer : The vinyl group enables polymerization via radical or cationic pathways. EPR studies show that this compound forms dialkoxyalkyl radicals during initiation, with β-scission rates influenced by the dioxolane ring’s steric constraints . Cationic ring-opening polymerization (e.g., using BF₃ catalysts) proceeds through oxonium ion intermediates, with ring strain (from the five-membered dioxolane) accelerating propagation .
Q. What solvent effects govern the hydrolysis kinetics of this compound derivatives, and how do they compare to analogous dioxanes?
- Answer : Hydrolysis rates (A-1 or A-Se₂ mechanisms) depend on solvent polarity. In water-dioxane mixtures, this compound exhibits similar solvent effects to 2-methyl derivatives, with transition-state stabilization via hydrogen bonding . Comparatively, dioxanes show slower hydrolysis due to reduced ring strain, highlighting the dioxolane’s enhanced reactivity .
Q. How can spectroscopic techniques (EPR, IR) resolve contradictions in radical intermediate stability during polymerization?
- Answer : EPR hyperfine splitting data (e.g., 69 G for this compound radicals) confirm the formation of allyl-type radicals, while IR (e.g., absence of ester peaks at 1730 cm⁻¹) rules out competing isomerization pathways . Discrepancies in reported rate constants for β-scission (e.g., 3.6 × 10⁶ s⁻¹ ) can be reconciled by comparing solvent and temperature conditions.
Q. What are the stability challenges of this compound under long-term storage, and how do degradation products impact analytical results?
- Answer : Migration studies in PET bottles detected trace 2-methyl-1,3-dioxolane (up to 19 ng/mL in ethanol), suggesting hydrolytic degradation of the vinyl group over time . Stability assessments should include GC-MS monitoring for acrolein (a hydrolysis byproduct) and storage under anhydrous conditions at ≤4°C .
Q. Methodological Considerations
Properties
IUPAC Name |
2-ethenyl-1,3-dioxolane | |
---|---|---|
Source | PubChem | |
URL | https://pubchem.ncbi.nlm.nih.gov | |
Description | Data deposited in or computed by PubChem | |
InChI |
InChI=1S/C5H8O2/c1-2-5-6-3-4-7-5/h2,5H,1,3-4H2 | |
Source | PubChem | |
URL | https://pubchem.ncbi.nlm.nih.gov | |
Description | Data deposited in or computed by PubChem | |
InChI Key |
KKBHSBATGOQADJ-UHFFFAOYSA-N | |
Source | PubChem | |
URL | https://pubchem.ncbi.nlm.nih.gov | |
Description | Data deposited in or computed by PubChem | |
Canonical SMILES |
C=CC1OCCO1 | |
Source | PubChem | |
URL | https://pubchem.ncbi.nlm.nih.gov | |
Description | Data deposited in or computed by PubChem | |
Molecular Formula |
C5H8O2 | |
Source | PubChem | |
URL | https://pubchem.ncbi.nlm.nih.gov | |
Description | Data deposited in or computed by PubChem | |
DSSTOX Substance ID |
DTXSID4073334 | |
Record name | 1,3-Dioxolane, 2-ethenyl- | |
Source | EPA DSSTox | |
URL | https://comptox.epa.gov/dashboard/DTXSID4073334 | |
Description | DSSTox provides a high quality public chemistry resource for supporting improved predictive toxicology. | |
Molecular Weight |
100.12 g/mol | |
Source | PubChem | |
URL | https://pubchem.ncbi.nlm.nih.gov | |
Description | Data deposited in or computed by PubChem | |
CAS No. |
3984-22-3 | |
Record name | 2-Ethenyl-1,3-dioxolane | |
Source | CAS Common Chemistry | |
URL | https://commonchemistry.cas.org/detail?cas_rn=3984-22-3 | |
Description | CAS Common Chemistry is an open community resource for accessing chemical information. Nearly 500,000 chemical substances from CAS REGISTRY cover areas of community interest, including common and frequently regulated chemicals, and those relevant to high school and undergraduate chemistry classes. This chemical information, curated by our expert scientists, is provided in alignment with our mission as a division of the American Chemical Society. | |
Explanation | The data from CAS Common Chemistry is provided under a CC-BY-NC 4.0 license, unless otherwise stated. | |
Record name | 1,3-Dioxolane, 2-ethenyl- | |
Source | ChemIDplus | |
URL | https://pubchem.ncbi.nlm.nih.gov/substance/?source=chemidplus&sourceid=0003984223 | |
Description | ChemIDplus is a free, web search system that provides access to the structure and nomenclature authority files used for the identification of chemical substances cited in National Library of Medicine (NLM) databases, including the TOXNET system. | |
Record name | 1,3-Dioxolane, 2-ethenyl- | |
Source | EPA Chemicals under the TSCA | |
URL | https://www.epa.gov/chemicals-under-tsca | |
Description | EPA Chemicals under the Toxic Substances Control Act (TSCA) collection contains information on chemicals and their regulations under TSCA, including non-confidential content from the TSCA Chemical Substance Inventory and Chemical Data Reporting. | |
Record name | 1,3-Dioxolane, 2-ethenyl- | |
Source | EPA DSSTox | |
URL | https://comptox.epa.gov/dashboard/DTXSID4073334 | |
Description | DSSTox provides a high quality public chemistry resource for supporting improved predictive toxicology. | |
Record name | 2-vinyl-1,3-dioxolane | |
Source | European Chemicals Agency (ECHA) | |
URL | https://echa.europa.eu/substance-information/-/substanceinfo/100.021.480 | |
Description | The European Chemicals Agency (ECHA) is an agency of the European Union which is the driving force among regulatory authorities in implementing the EU's groundbreaking chemicals legislation for the benefit of human health and the environment as well as for innovation and competitiveness. | |
Explanation | Use of the information, documents and data from the ECHA website is subject to the terms and conditions of this Legal Notice, and subject to other binding limitations provided for under applicable law, the information, documents and data made available on the ECHA website may be reproduced, distributed and/or used, totally or in part, for non-commercial purposes provided that ECHA is acknowledged as the source: "Source: European Chemicals Agency, http://echa.europa.eu/". Such acknowledgement must be included in each copy of the material. ECHA permits and encourages organisations and individuals to create links to the ECHA website under the following cumulative conditions: Links can only be made to webpages that provide a link to the Legal Notice page. | |
Retrosynthesis Analysis
AI-Powered Synthesis Planning: Our tool employs the Template_relevance Pistachio, Template_relevance Bkms_metabolic, Template_relevance Pistachio_ringbreaker, Template_relevance Reaxys, Template_relevance Reaxys_biocatalysis model, leveraging a vast database of chemical reactions to predict feasible synthetic routes.
One-Step Synthesis Focus: Specifically designed for one-step synthesis, it provides concise and direct routes for your target compounds, streamlining the synthesis process.
Accurate Predictions: Utilizing the extensive PISTACHIO, BKMS_METABOLIC, PISTACHIO_RINGBREAKER, REAXYS, REAXYS_BIOCATALYSIS database, our tool offers high-accuracy predictions, reflecting the latest in chemical research and data.
Strategy Settings
Precursor scoring | Relevance Heuristic |
---|---|
Min. plausibility | 0.01 |
Model | Template_relevance |
Template Set | Pistachio/Bkms_metabolic/Pistachio_ringbreaker/Reaxys/Reaxys_biocatalysis |
Top-N result to add to graph | 6 |
Feasible Synthetic Routes
Disclaimer and Information on In-Vitro Research Products
Please be aware that all articles and product information presented on BenchChem are intended solely for informational purposes. The products available for purchase on BenchChem are specifically designed for in-vitro studies, which are conducted outside of living organisms. In-vitro studies, derived from the Latin term "in glass," involve experiments performed in controlled laboratory settings using cells or tissues. It is important to note that these products are not categorized as medicines or drugs, and they have not received approval from the FDA for the prevention, treatment, or cure of any medical condition, ailment, or disease. We must emphasize that any form of bodily introduction of these products into humans or animals is strictly prohibited by law. It is essential to adhere to these guidelines to ensure compliance with legal and ethical standards in research and experimentation.