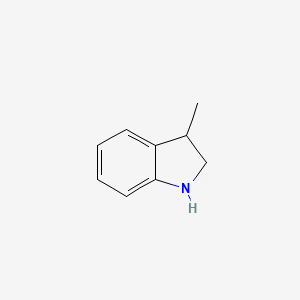
3-Methylindoline
Overview
Description
3-Methylindoline, also known as 3-methyl-1H-indole, is an organic compound belonging to the indole family. It is a derivative of indole with a methyl group attached to the third carbon of the indole ring. This compound is known for its presence in various natural sources and its significance in both biological and industrial applications.
Preparation Methods
Synthetic Routes and Reaction Conditions: 3-Methylindoline can be synthesized through several methods. One common approach is the Fischer indole synthesis, which involves the reaction of phenylhydrazine with ketones or aldehydes under acidic conditions. Another method involves the reduction of indole-3-carboxaldehyde derivatives using hydrogenation catalysts such as copper or copper oxide-based catalysts .
Industrial Production Methods: In industrial settings, this compound is often produced through the hydrogenation of indole-3-carboxaldehyde derivatives. This process is performed in the presence of a solvent under conditions of 50-300°C and 50-1,000 psig with a hydrogen atmosphere . The use of relatively inexpensive hydrogenation catalysts makes this method economically viable.
Chemical Reactions Analysis
Types of Reactions: 3-Methylindoline undergoes various chemical reactions, including oxidation, reduction, and substitution reactions.
Common Reagents and Conditions:
Oxidation: this compound can be oxidized using reagents such as potassium permanganate or chromium trioxide to form corresponding indole-3-carboxylic acids.
Reduction: Reduction of this compound can be achieved using hydrogenation catalysts like palladium on carbon or copper-based catalysts.
Substitution: Electrophilic substitution reactions can occur at the indole ring, particularly at the nitrogen atom or the carbon atoms adjacent to the nitrogen.
Major Products:
Oxidation Products: Indole-3-carboxylic acids.
Reduction Products: Reduced forms of this compound, such as this compound derivatives.
Substitution Products: Various substituted indole derivatives depending on the electrophile used.
Scientific Research Applications
3-Methylindoline has a wide range of applications in scientific research, including:
Chemistry:
- Used as a building block in the synthesis of more complex indole derivatives.
- Employed in the study of reaction mechanisms and the development of new synthetic methodologies.
Biology:
- Investigated for its role in biological systems, particularly in the metabolism of tryptophan.
- Studied for its potential effects on human health, including its cytotoxicity to bronchial epithelial cells .
Medicine:
- Explored for its potential therapeutic applications, including its use as a precursor in the synthesis of pharmaceutical compounds.
- Investigated for its role in the development of new drugs targeting specific biological pathways.
Industry:
- Used in the production of fragrances and perfumes due to its distinct odor.
- Employed as an intermediate in the synthesis of agrochemicals and other industrial chemicals.
Mechanism of Action
The mechanism of action of 3-Methylindoline involves its interaction with various molecular targets and pathways. In biological systems, it is metabolized by cytochrome P450 enzymes, leading to the formation of reactive metabolites such as 3-methyleneindolenine . These metabolites can induce cytotoxicity and apoptosis in cells by interacting with cellular macromolecules and disrupting normal cellular functions.
Comparison with Similar Compounds
Indole: The parent compound of 3-Methylindoline, known for its presence in various natural sources and its role in biological processes.
3-Methylindole: A closely related compound with a similar structure but different chemical properties and applications.
Indole-3-acetic acid: A plant hormone derived from indole, known for its role in plant growth and development.
Uniqueness of this compound:
- The presence of a methyl group at the third carbon of the indole ring imparts unique chemical properties to this compound, making it distinct from other indole derivatives.
- Its specific reactivity and applications in various fields, including chemistry, biology, and industry, highlight its importance as a versatile compound.
Properties
IUPAC Name |
3-methyl-2,3-dihydro-1H-indole | |
---|---|---|
Source | PubChem | |
URL | https://pubchem.ncbi.nlm.nih.gov | |
Description | Data deposited in or computed by PubChem | |
InChI |
InChI=1S/C9H11N/c1-7-6-10-9-5-3-2-4-8(7)9/h2-5,7,10H,6H2,1H3 | |
Source | PubChem | |
URL | https://pubchem.ncbi.nlm.nih.gov | |
Description | Data deposited in or computed by PubChem | |
InChI Key |
BFQARNDIMKOOQQ-UHFFFAOYSA-N | |
Source | PubChem | |
URL | https://pubchem.ncbi.nlm.nih.gov | |
Description | Data deposited in or computed by PubChem | |
Canonical SMILES |
CC1CNC2=CC=CC=C12 | |
Source | PubChem | |
URL | https://pubchem.ncbi.nlm.nih.gov | |
Description | Data deposited in or computed by PubChem | |
Molecular Formula |
C9H11N | |
Source | PubChem | |
URL | https://pubchem.ncbi.nlm.nih.gov | |
Description | Data deposited in or computed by PubChem | |
DSSTOX Substance ID |
DTXSID50335319 | |
Record name | 3-methylindoline | |
Source | EPA DSSTox | |
URL | https://comptox.epa.gov/dashboard/DTXSID50335319 | |
Description | DSSTox provides a high quality public chemistry resource for supporting improved predictive toxicology. | |
Molecular Weight |
133.19 g/mol | |
Source | PubChem | |
URL | https://pubchem.ncbi.nlm.nih.gov | |
Description | Data deposited in or computed by PubChem | |
CAS No. |
4375-15-9 | |
Record name | 3-Methylindoline | |
Source | CAS Common Chemistry | |
URL | https://commonchemistry.cas.org/detail?cas_rn=4375-15-9 | |
Description | CAS Common Chemistry is an open community resource for accessing chemical information. Nearly 500,000 chemical substances from CAS REGISTRY cover areas of community interest, including common and frequently regulated chemicals, and those relevant to high school and undergraduate chemistry classes. This chemical information, curated by our expert scientists, is provided in alignment with our mission as a division of the American Chemical Society. | |
Explanation | The data from CAS Common Chemistry is provided under a CC-BY-NC 4.0 license, unless otherwise stated. | |
Record name | 3-methylindoline | |
Source | EPA DSSTox | |
URL | https://comptox.epa.gov/dashboard/DTXSID50335319 | |
Description | DSSTox provides a high quality public chemistry resource for supporting improved predictive toxicology. | |
Synthesis routes and methods I
Procedure details
Synthesis routes and methods II
Procedure details
Retrosynthesis Analysis
AI-Powered Synthesis Planning: Our tool employs the Template_relevance Pistachio, Template_relevance Bkms_metabolic, Template_relevance Pistachio_ringbreaker, Template_relevance Reaxys, Template_relevance Reaxys_biocatalysis model, leveraging a vast database of chemical reactions to predict feasible synthetic routes.
One-Step Synthesis Focus: Specifically designed for one-step synthesis, it provides concise and direct routes for your target compounds, streamlining the synthesis process.
Accurate Predictions: Utilizing the extensive PISTACHIO, BKMS_METABOLIC, PISTACHIO_RINGBREAKER, REAXYS, REAXYS_BIOCATALYSIS database, our tool offers high-accuracy predictions, reflecting the latest in chemical research and data.
Strategy Settings
Precursor scoring | Relevance Heuristic |
---|---|
Min. plausibility | 0.01 |
Model | Template_relevance |
Template Set | Pistachio/Bkms_metabolic/Pistachio_ringbreaker/Reaxys/Reaxys_biocatalysis |
Top-N result to add to graph | 6 |
Feasible Synthetic Routes
Q1: What makes 3-methylindoline significant in biological research?
A: this compound is a key intermediate in the metabolic pathway of 3-methylindole, a pneumotoxic compound found in various environments. Understanding the formation and fate of this compound is crucial due to its potential role in 3-methylindole toxicity. [, ]
Q2: How is this compound formed in biological systems?
A: Research suggests that this compound is formed through the cytochrome P450-mediated epoxidation of 3-methylindole. This process leads to the formation of 2,3-epoxy-3-methylindoline, a reactive intermediate. This epoxide can then undergo ring opening and a hydride shift to form this compound. Evidence for this pathway includes the retention of deuterium from labeled 3-methylindole during in vitro studies with goat lung microsomes. [, ]
Q3: What are the potential reactive intermediates formed during this compound formation?
A: Studies utilizing stable isotope techniques and mass spectrometry have identified two key reactive intermediates: [, ]
Q4: Can you elaborate on the experimental evidence supporting the formation of these reactive intermediates?
A: Several key findings support the existence of these intermediates: [, ]
Q5: Beyond its role in 3-methylindole metabolism, what other research has been conducted on this compound?
A: this compound derivatives have shown potential as inhibitors of EZH2, an enzyme implicated in certain cancers. Researchers have explored the design, synthesis, and structure-activity relationships of novel this compound-based EZH2 inhibitors. [] Additionally, this compound derivatives have been synthesized and studied for their selective toxicity against the SH-SY5Y cell line. []
Q6: What are the potential applications of this research in drug development?
A: While this compound itself might not be a direct drug candidate, understanding its formation and reactivity is crucial for: [, , ]
Disclaimer and Information on In-Vitro Research Products
Please be aware that all articles and product information presented on BenchChem are intended solely for informational purposes. The products available for purchase on BenchChem are specifically designed for in-vitro studies, which are conducted outside of living organisms. In-vitro studies, derived from the Latin term "in glass," involve experiments performed in controlled laboratory settings using cells or tissues. It is important to note that these products are not categorized as medicines or drugs, and they have not received approval from the FDA for the prevention, treatment, or cure of any medical condition, ailment, or disease. We must emphasize that any form of bodily introduction of these products into humans or animals is strictly prohibited by law. It is essential to adhere to these guidelines to ensure compliance with legal and ethical standards in research and experimentation.