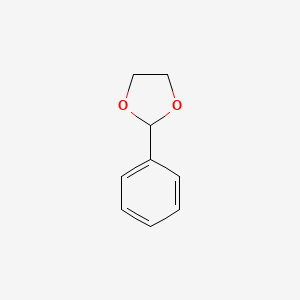
2-Phenyl-1,3-dioxolane
Overview
Description
2-Phenyl-1,3-dioxolane (CAS 936-51-6, EC 213-315-5) is a five-membered cyclic acetal characterized by a phenyl group substituted at the 2-position of the 1,3-dioxolane ring. It is widely utilized as a protecting group for carbonyl compounds and alcohols in organic synthesis due to its stability under acidic and oxidative conditions . Key synthetic methods include:
- Catalytic acetalization: Copper phosphotungstate catalyzes its formation from benzaldehyde and ethylene glycol with 66.7% yield under optimized conditions (0.5 g catalyst, 2 h reflux) .
- I₂/PAn catalysis: Achieves yields up to 94.2% under mild conditions (1:1.6 aldehyde/glycol ratio, 1 h reaction time) .
- Ring-opening reactivity: Reacts with Lewis acids (e.g., BF₃) to yield ethylene glycol benzyl ethers and hydroxyethyl benzoate (90% yield) .
Its stereoelectronic properties are influenced by hyperconjugation between the C–H bonds and the aromatic ring, with a dihedral angle of ~80° between H1 and the phenyl group, as determined by DFT studies .
Preparation Methods
Preparation Methods
Synthesis via Acetal Halides (Radical Ring-Opening Polymerization Precursors)
A notable method for preparing 2-phenyl-1,3-dioxolane derivatives involves the synthesis of 2-methylene-4-phenyl-1,3-dioxolane (a related cyclic ketene acetal) from various acetal halides. This approach was detailed in a 2016 study, which reported:
- Use of three different acetal halides as starting materials.
- Characterization of the product by $$ ^1H $$ and $$ ^{13}C $$ NMR spectroscopy, IR spectroscopy, elemental analysis, and mass spectrometry.
- The product serves as a monomer for radical ring-opening polymerization, enabling the production of degradable polymethacrylates with tunable properties.
This method highlights the versatility of this compound derivatives in polymer chemistry and the importance of selecting appropriate halide precursors to optimize synthesis efficiency.
Reduction of Diethyl Phenylmalonate to 2-Phenyl-1,3-propanediol (Related Intermediate)
Although this method targets 2-phenyl-1,3-propanediol, it is relevant because this intermediate can be converted into this compound derivatives. The process involves:
- Reaction of diethyl phenylmalonate with sodium borohydride in the presence of an alkali metal dihydrogen phosphate buffer.
- Use of polar solvents such as ethanol, tetrahydrofuran (THF), or dioxane.
- Maintaining pH between 5.0 and 6.0 to optimize yield and minimize impurities.
- The molar ratio of sodium dihydrogen phosphate to sodium borohydride is preferably 1:1.
- The process yields 60-70% of 2-phenyl-1,3-propanediol with impurity levels below 1%.
- This method is advantageous due to reduced hazards and cost-effectiveness compared to traditional hydride reductions using borane or lithium aluminum hydride.
The reaction scheme includes quenching, basifying, and organic solvent extraction steps to isolate the product.
Multi-Step Reduction Using Zinc/Aluminum Powder and Tetrabutylammonium Chloride
Another reported method for synthesizing 2-phenyl-1,3-propanediol (a precursor to dioxolane derivatives) involves:
- Reduction of diethyl 2-phenylmalonate under argon atmosphere.
- Use of a reducing agent prepared from zinc powder, aluminum powder, and activated carbon in acetic acid.
- Reaction temperature controlled between 145°C and 156°C under increased pressure (3-5 atmospheres).
- Sequential addition of sodium hydroxide solution and tetrabutylammonium chloride as phase transfer catalyst.
- Use of tetrahydrofuran (THF) and toluene as solvents.
- After reaction completion, the mixture is cooled, filtered, washed, dried, and concentrated to yield the product.
- This method achieves a molar yield of 99.6% with gas chromatography purity of 99.3%.
While focused on the diol, this high-purity intermediate can be cyclized to form this compound under suitable conditions.
Comparative Data Table of Preparation Methods
Chemical Reactions Analysis
Types of Reactions: 2-Phenyl-1,3-dioxolane undergoes various chemical reactions, including:
Reduction: Reduction can be achieved using agents like lithium aluminum hydride (LiAlH4) or sodium borohydride (NaBH4).
Substitution: It can undergo nucleophilic substitution reactions with reagents like Grignard reagents (RMgX) or organolithium compounds (RLi).
Common Reagents and Conditions:
Oxidation: KMnO4, CrO3, OsO4
Reduction: LiAlH4, NaBH4
Substitution: RMgX, RLi
Major Products Formed:
Oxidation: Benzaldehyde
Reduction: Corresponding alcohols
Substitution: Various substituted phenyl derivatives.
Scientific Research Applications
Flavor and Fragrance Applications
2-Phenyl-1,3-dioxolane and its derivatives are primarily utilized in the flavor and fragrance sectors. They are known for imparting desirable sensory experiences in various consumer products.
Cooling Sensation in Products
The compound is incorporated into products such as:
- Toothpastes
- Mouthwashes
- Chewing gums
- Beverages
These products benefit from the compound's ability to create a cooling sensation, which enhances the user experience. Research indicates that derivatives like 4-(1-menthoxymethyl)-2-phenyl-1,3-dioxolane can significantly prolong this sensation when combined with menthol and other flavoring agents .
Cosmetic and Pharmaceutical Uses
In cosmetics and pharmaceuticals, this compound is used in products such as:
- Deodorants
- Perfumes
- Soaps
The compound's properties allow for improved stability and longevity of fragrances in these formulations .
Chemical Reactions Involving this compound
The compound also plays a significant role in various chemical reactions, particularly in organic synthesis.
Base-Induced Fragmentation
A study highlighted the base-induced fragmentation of this compound, which can lead to the formation of valuable intermediates for further chemical transformations. This process has implications for synthetic organic chemistry where such intermediates can be utilized for creating more complex molecules .
Acetalization Reactions
Research has demonstrated that this compound can participate in acetalization reactions with carbonyl compounds like benzaldehyde and furfural. The resulting products include various dioxolanes and dioxanes, which are essential in synthesizing other organic compounds .
Photochemical Applications
The photochemical properties of this compound have been studied for their potential in polymerization processes.
Photoinitiators in Polymer Chemistry
The compound has been investigated as a component of photoinitiators used in free radical polymerization processes. These systems often involve light-sensitive components that initiate polymerization upon exposure to UV or visible light .
Flavor Composition Studies
In one study, various concentrations of 4-(1-menthoxymethyl)-2-phenyl-1,3-dioxolane were tested in flavor compositions for confectionery products. The results indicated optimal concentrations ranged from 0.001% to 10%, achieving a balance between flavor intensity and sensory experience .
Product Type | Concentration Range (%) | Sensory Effect |
---|---|---|
Chewing Gum | 0.001 - 10 | Cooling sensation |
Mouthwash | 0.0001 - 10 | Long-lasting flavor |
Perfumes | 0.0001 - 30 | Enhanced fragrance stability |
Acetalization Reaction Outcomes
A series of acetalization reactions involving benzaldehyde demonstrated the versatility of this compound as a reagent:
Reaction Type | Catalyst Used | Reaction Time (hours) | Product Yield (%) |
---|---|---|---|
Acetalization with Benzaldehyde | Al-SBA-15 | 8 | 93 |
Acetalization with Furfural | Al-SBA-15 | 12 | Varied |
Mechanism of Action
The mechanism of action of 2-Phenyl-1,3-dioxolane primarily involves its role as a protecting group. It forms a stable cyclic acetal with carbonyl compounds, thereby preventing them from undergoing reactions. This stability is due to the formation of a five-membered ring, which is less prone to hydrolysis compared to other protecting groups .
Comparison with Similar Compounds
Structural and Electronic Properties
Key Findings :
- The oxygen atoms in this compound induce a near-orthogonal H1 orientation, enhancing hyperconjugation compared to sulfur in dithiane derivatives .
- Six-membered analogues (e.g., 2-phenyl-1,3-dioxane) exhibit lower ring strain but form less readily in acetalization reactions .
Key Findings :
- Electron-donating groups (e.g., methyl) improve yields in acetalization but hinder ring-opening reactions due to steric effects .
- Electron-withdrawing substituents (e.g., nitro) alter electronic properties but are less explored in industrial applications .
Key Findings :
Biological Activity
2-Phenyl-1,3-dioxolane is a heterocyclic organic compound characterized by a five-membered ring containing two oxygen atoms. Its molecular formula is , with a molecular weight of approximately 150.18 g/mol. This compound has garnered attention in the field of medicinal chemistry due to its potential biological activities, including antimicrobial and anticancer properties.
Antimicrobial Properties
Recent studies have highlighted the potential of this compound derivatives as antimicrobial agents. For instance, research conducted on various dioxolane compounds demonstrated significant antibacterial activity against a range of pathogens. The structure-activity relationship (SAR) analysis indicated that modifications to the phenyl ring could enhance biological activity, particularly through the introduction of electron-withdrawing groups which increase reactivity towards biological targets .
Anticancer Activity
In addition to its antimicrobial properties, this compound has been investigated for its anticancer potential. Studies have shown that certain derivatives exhibit cytotoxic effects on cancer cell lines. The mechanism of action appears to involve the induction of apoptosis and the inhibition of cell proliferation. Notably, compounds with specific substituents on the phenyl ring demonstrated enhanced potency against various cancer types .
The biological activity of this compound is believed to stem from its ability to interact with cellular enzymes and receptors. The presence of the dioxolane ring structure allows for versatile interactions with nucleophiles and electrophiles within biological systems. This reactivity can lead to the modification of biomolecules such as proteins and nucleic acids, ultimately affecting cellular processes .
Study 1: Antimicrobial Efficacy
A study evaluated the antimicrobial efficacy of several dioxolane derivatives, including this compound. The results indicated that this compound exhibited significant inhibitory effects against Staphylococcus aureus and Escherichia coli. The minimum inhibitory concentration (MIC) values were determined, showing that modifications to the dioxolane structure could enhance activity:
Compound | MIC (µg/mL) | Target Pathogen |
---|---|---|
This compound | 32 | S. aureus |
4-Nitrophenyl derivative | 16 | E. coli |
Study 2: Anticancer Activity
In another study focusing on anticancer properties, various derivatives of this compound were tested against human cancer cell lines. The following table summarizes the findings:
Compound | IC50 (µM) | Cancer Cell Line |
---|---|---|
This compound | 25 | HeLa (cervical cancer) |
Methyl-substituted derivative | 15 | MCF-7 (breast cancer) |
These results indicate that certain modifications can significantly enhance the anticancer activity of dioxolanes.
Q & A
Q. What are the established synthetic methodologies for preparing 2-Phenyl-1,3-dioxolane in laboratory settings?
The synthesis of this compound primarily involves acid-catalyzed acetalization of benzaldehyde with glycerol or its derivatives. A green chemistry approach utilizes heterogeneous catalysts, such as sulfamic acid-functionalized magnetic nanoparticles (SA-MNPs), to improve reaction efficiency and enable catalyst recyclability. Key parameters include temperature control (80–120°C), solvent-free conditions, and continuous flow reactors for optimized mixing and heat transfer, achieving yields >85% .
Q. How is the structural conformation of this compound analyzed to predict its reactivity?
Structural analysis employs spectroscopic techniques (¹H/¹³C NMR, IR) and computational modeling. Density Functional Theory (DFT) at the B3LYP/6-31G* level calculates dihedral angles between the dioxolane ring and phenyl group (≈80°), revealing near-orthogonal alignment. This steric arrangement suppresses sp³ C-O cleavage under standard conditions, as confirmed by hyperconjugation analysis between C-H bonds and the aromatic ring .
Q. How can researchers resolve contradictions in reported reactivity of this compound toward C-O bond cleavage?
Discrepancies in reactivity (e.g., failed vs. low-yield cleavage) require systematic experimental design:
- Parameter Variation : Test catalysts (e.g., Lewis acids, transition metals), solvents (polar vs. nonpolar), and temperatures.
- Computational Validation : Use DFT to model transition states and identify conformational flexibility requirements.
For example, rigid five-membered dioxolane rings lack the flexibility needed for cleavage, unlike larger analogs (e.g., 1,3-dioxanes) .
Q. What computational strategies are employed to elucidate the electronic properties of this compound?
Quantum mechanical methods (DFT-B3LYP/6-31G*) optimize geometry and evaluate electronic effects:
- Hyperconjugation : Assess interactions between σ(C-H) orbitals and π*(aromatic ring) to explain stability.
- Frontier Molecular Orbitals (FMOs) : Analyze HOMO-LUMO gaps to predict redox behavior.
These models guide synthetic modifications, such as fluorination, to enhance lipophilicity or biological activity .
Q. What pharmacological relevance does this compound hold in medicinal chemistry?
This compound derivatives are precursors for CNS-active compounds. For instance, irradiation of related structures yields benzodiazepine analogs with anticonvulsant properties. Its dioxolane ring enhances metabolic stability, making it a scaffold for neuroactive drug candidates .
Q. What experimental approaches optimize the sustainability of this compound synthesis?
Sustainable synthesis strategies include:
- Heterogeneous Catalysis : Acidic resins or SA-MNPs reduce waste and enable catalyst reuse.
- Process Intensification : Automated continuous flow reactors improve heat/mass transfer and scalability.
- Solvent-Free Conditions : Minimize environmental impact while maintaining >90% conversion .
Q. How does substituent variation on the phenyl ring influence the biological activity of this compound derivatives?
Comparative studies show:
- Fluorination : Introducing 3,4-difluoro groups increases lipophilicity and antimicrobial activity.
- Halogenation : Bromo or chloro substituents enhance electrophilicity, improving reactivity in cross-coupling reactions.
Structure-activity relationship (SAR) tables guide targeted modifications for specific biological endpoints (e.g., anticonvulsant vs. antimicrobial) .
Q. What mechanistic insights explain the resistance of this compound to radical-initiated polymerization?
The near-orthogonal phenyl-dioxolane conformation sterically hinders radical attack at the C-O bond. Computational models (DFT) confirm higher activation energies for ring-opening compared to analogs like 2-vinyl-1,3-dioxolane, which polymerize readily via radical pathways .
Q. How are stereoelectronic effects in this compound exploited in asymmetric catalysis?
The dioxolane ring’s electron-donating oxygen atoms stabilize transition metals (e.g., Rh, Pd) in chiral complexes. For example, (+)-4,5-bis(hydroxydiphenylmethyl) derivatives act as ligands, inducing enantioselectivity (>90% ee) in hydrogenation reactions .
Q. What analytical challenges arise in characterizing this compound derivatives, and how are they addressed?
Challenges :
- Overlapping NMR signals due to symmetry.
- Low volatility for GC-MS analysis.
Solutions : - Use high-field NMR (600 MHz) with 2D-COSY for signal assignment.
- Derivatization (e.g., silylation) improves volatility for mass spectrometry .
Properties
IUPAC Name |
2-phenyl-1,3-dioxolane | |
---|---|---|
Source | PubChem | |
URL | https://pubchem.ncbi.nlm.nih.gov | |
Description | Data deposited in or computed by PubChem | |
InChI |
InChI=1S/C9H10O2/c1-2-4-8(5-3-1)9-10-6-7-11-9/h1-5,9H,6-7H2 | |
Source | PubChem | |
URL | https://pubchem.ncbi.nlm.nih.gov | |
Description | Data deposited in or computed by PubChem | |
InChI Key |
LYINTWKRUWVLBA-UHFFFAOYSA-N | |
Source | PubChem | |
URL | https://pubchem.ncbi.nlm.nih.gov | |
Description | Data deposited in or computed by PubChem | |
Canonical SMILES |
C1COC(O1)C2=CC=CC=C2 | |
Source | PubChem | |
URL | https://pubchem.ncbi.nlm.nih.gov | |
Description | Data deposited in or computed by PubChem | |
Molecular Formula |
C9H10O2 | |
Source | PubChem | |
URL | https://pubchem.ncbi.nlm.nih.gov | |
Description | Data deposited in or computed by PubChem | |
DSSTOX Substance ID |
DTXSID00239479 | |
Record name | 2-Phenyl-1,3-dioxolane | |
Source | EPA DSSTox | |
URL | https://comptox.epa.gov/dashboard/DTXSID00239479 | |
Description | DSSTox provides a high quality public chemistry resource for supporting improved predictive toxicology. | |
Molecular Weight |
150.17 g/mol | |
Source | PubChem | |
URL | https://pubchem.ncbi.nlm.nih.gov | |
Description | Data deposited in or computed by PubChem | |
CAS No. |
936-51-6 | |
Record name | 2-Phenyl-1,3-dioxolane | |
Source | CAS Common Chemistry | |
URL | https://commonchemistry.cas.org/detail?cas_rn=936-51-6 | |
Description | CAS Common Chemistry is an open community resource for accessing chemical information. Nearly 500,000 chemical substances from CAS REGISTRY cover areas of community interest, including common and frequently regulated chemicals, and those relevant to high school and undergraduate chemistry classes. This chemical information, curated by our expert scientists, is provided in alignment with our mission as a division of the American Chemical Society. | |
Explanation | The data from CAS Common Chemistry is provided under a CC-BY-NC 4.0 license, unless otherwise stated. | |
Record name | 2-Phenyl-1,3-dioxolane | |
Source | ChemIDplus | |
URL | https://pubchem.ncbi.nlm.nih.gov/substance/?source=chemidplus&sourceid=0000936516 | |
Description | ChemIDplus is a free, web search system that provides access to the structure and nomenclature authority files used for the identification of chemical substances cited in National Library of Medicine (NLM) databases, including the TOXNET system. | |
Record name | 2-Phenyl-1,3-dioxolane | |
Source | DTP/NCI | |
URL | https://dtp.cancer.gov/dtpstandard/servlet/dwindex?searchtype=NSC&outputformat=html&searchlist=97528 | |
Description | The NCI Development Therapeutics Program (DTP) provides services and resources to the academic and private-sector research communities worldwide to facilitate the discovery and development of new cancer therapeutic agents. | |
Explanation | Unless otherwise indicated, all text within NCI products is free of copyright and may be reused without our permission. Credit the National Cancer Institute as the source. | |
Record name | 2-Phenyl-1,3-dioxolane | |
Source | EPA DSSTox | |
URL | https://comptox.epa.gov/dashboard/DTXSID00239479 | |
Description | DSSTox provides a high quality public chemistry resource for supporting improved predictive toxicology. | |
Record name | 2-phenyl-1,3-dioxolane | |
Source | European Chemicals Agency (ECHA) | |
URL | https://echa.europa.eu/substance-information/-/substanceinfo/100.012.104 | |
Description | The European Chemicals Agency (ECHA) is an agency of the European Union which is the driving force among regulatory authorities in implementing the EU's groundbreaking chemicals legislation for the benefit of human health and the environment as well as for innovation and competitiveness. | |
Explanation | Use of the information, documents and data from the ECHA website is subject to the terms and conditions of this Legal Notice, and subject to other binding limitations provided for under applicable law, the information, documents and data made available on the ECHA website may be reproduced, distributed and/or used, totally or in part, for non-commercial purposes provided that ECHA is acknowledged as the source: "Source: European Chemicals Agency, http://echa.europa.eu/". Such acknowledgement must be included in each copy of the material. ECHA permits and encourages organisations and individuals to create links to the ECHA website under the following cumulative conditions: Links can only be made to webpages that provide a link to the Legal Notice page. | |
Synthesis routes and methods I
Procedure details
Synthesis routes and methods II
Procedure details
Synthesis routes and methods III
Procedure details
Retrosynthesis Analysis
AI-Powered Synthesis Planning: Our tool employs the Template_relevance Pistachio, Template_relevance Bkms_metabolic, Template_relevance Pistachio_ringbreaker, Template_relevance Reaxys, Template_relevance Reaxys_biocatalysis model, leveraging a vast database of chemical reactions to predict feasible synthetic routes.
One-Step Synthesis Focus: Specifically designed for one-step synthesis, it provides concise and direct routes for your target compounds, streamlining the synthesis process.
Accurate Predictions: Utilizing the extensive PISTACHIO, BKMS_METABOLIC, PISTACHIO_RINGBREAKER, REAXYS, REAXYS_BIOCATALYSIS database, our tool offers high-accuracy predictions, reflecting the latest in chemical research and data.
Strategy Settings
Precursor scoring | Relevance Heuristic |
---|---|
Min. plausibility | 0.01 |
Model | Template_relevance |
Template Set | Pistachio/Bkms_metabolic/Pistachio_ringbreaker/Reaxys/Reaxys_biocatalysis |
Top-N result to add to graph | 6 |
Feasible Synthetic Routes
Disclaimer and Information on In-Vitro Research Products
Please be aware that all articles and product information presented on BenchChem are intended solely for informational purposes. The products available for purchase on BenchChem are specifically designed for in-vitro studies, which are conducted outside of living organisms. In-vitro studies, derived from the Latin term "in glass," involve experiments performed in controlled laboratory settings using cells or tissues. It is important to note that these products are not categorized as medicines or drugs, and they have not received approval from the FDA for the prevention, treatment, or cure of any medical condition, ailment, or disease. We must emphasize that any form of bodily introduction of these products into humans or animals is strictly prohibited by law. It is essential to adhere to these guidelines to ensure compliance with legal and ethical standards in research and experimentation.