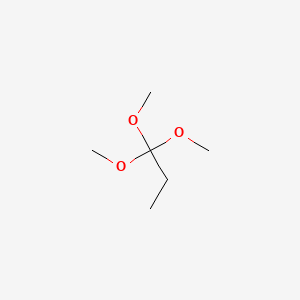
Trimethyl orthopropionate
Overview
Description
Trimethyl orthopropionate, also known as 1,1,1-trimethoxypropane, is an organic compound with the molecular formula C6H14O3. It is a clear, colorless liquid with a molecular weight of 134.17 g/mol. This compound is a member of the orthoester family, characterized by three alkoxy groups attached to a central carbon atom. This compound is used in various organic synthesis reactions due to its reactivity and versatility .
Mechanism of Action
Target of Action
Trimethyl orthopropionate is a chemical compound used as a substrate in various organic transformations . It is a functional group containing three alkoxy groups attached to one central carbon atom . The primary targets of this compound are the reactants in these transformations, which it interacts with to form new compounds .
Mode of Action
This compound interacts with its targets through a process known as nucleophilic substitution . This is a chemical reaction in which an electron-rich nucleophile selectively bonds with or attacks the positive or partially positive charge of an atom or a group of atoms to replace a leaving group; the positive or partially positive atom is referred to as the electrophile .
Biochemical Pathways
The biochemical pathways affected by this compound are those involved in the organic transformations for which it is used as a substrate . These transformations can involve various classes of two-component and multi-component organic reactions . The downstream effects of these pathways are the formation of new organic compounds .
Pharmacokinetics
It is known that this compound is a clear colorless liquid that is soluble in alcohol, chloroform, ethyl acetate, and ether, and it hydrolyzes slowly in water . These properties can influence its bioavailability in different environments.
Result of Action
The result of this compound’s action is the formation of new organic compounds through various classes of two-component and multi-component organic reactions . The specific molecular and cellular effects depend on the nature of the organic transformations in which it is used as a substrate .
Action Environment
The action of this compound can be influenced by environmental factors such as the reaction medium. For example, it can perform various classes of two-component and multi-component organic reactions under solvent-free conditions, in aqueous media, and in organic solvents . These conditions can influence the compound’s action, efficacy, and stability .
Preparation Methods
Synthetic Routes and Reaction Conditions: Trimethyl orthopropionate can be synthesized through the reaction of propionic acid with methanol in the presence of an acid catalyst. The reaction proceeds via the formation of an intermediate ester, which is then converted to the orthoester by further reaction with methanol. The general reaction scheme is as follows:
CH3CH2COOH+3CH3OH→CH3CH2C(OCH3)3+H2O
Industrial Production Methods: In industrial settings, the production of this compound typically involves continuous processes where propionic acid and methanol are fed into a reactor containing an acid catalyst. The reaction mixture is then distilled to separate the desired orthoester from by-products and unreacted starting materials. The use of azeotropic distillation can help in removing water formed during the reaction, driving the equilibrium towards the formation of the orthoester .
Chemical Reactions Analysis
Types of Reactions: Trimethyl orthopropionate undergoes various types of chemical reactions, including:
Hydrolysis: In the presence of water, this compound hydrolyzes to form propionic acid and methanol.
Transesterification: It can react with other alcohols to form different orthoesters.
Nucleophilic Substitution: The alkoxy groups can be replaced by nucleophiles such as amines or thiols.
Common Reagents and Conditions:
Hydrolysis: Acidic or basic conditions, water.
Transesterification: Alcohols, acid or base catalysts.
Nucleophilic Substitution: Nucleophiles (e.g., amines, thiols), solvents like ethanol or methanol.
Major Products Formed:
Hydrolysis: Propionic acid and methanol.
Transesterification: Different orthoesters depending on the alcohol used.
Nucleophilic Substitution: Substituted orthoesters with the nucleophile replacing one or more alkoxy groups
Scientific Research Applications
Trimethyl orthopropionate has several applications in scientific research:
Organic Synthesis: It is used as a reagent in the synthesis of various organic compounds, including pharmaceuticals and agrochemicals.
Polymer Chemistry: It serves as a monomer or a cross-linking agent in the production of polymers and resins.
Material Science: It is used in the preparation of advanced materials, such as coatings and adhesives, due to its reactivity and ability to form stable bonds.
Biological Studies: It is employed in the modification of biomolecules and the synthesis of biologically active compounds
Comparison with Similar Compounds
Trimethyl orthopropionate can be compared with other orthoesters, such as:
Trimethyl orthoformate (C4H10O3): Similar in structure but with a formate group instead of a propionate group. It is used in similar reactions but has different reactivity due to the formate group.
Triethyl orthoformate (C7H16O3): Contains ethyl groups instead of methyl groups, leading to different physical properties and reactivity.
Triethyl orthoacetate (C8H18O3): Similar to this compound but with an acetate group. It is used in different synthetic applications due to its unique reactivity.
The uniqueness of this compound lies in its specific reactivity profile and the ability to form stable products in various chemical reactions .
Biological Activity
Trimethyl orthopropionate (TMOP) is an organo-chemical compound that has garnered attention for its diverse applications in organic synthesis, polymer production, and research in chemical biology. This article delves into the biological activity of TMOP, highlighting its roles as a solvent, precursor for synthesis, and its implications in drug discovery and development.
Chemical Structure:
this compound is an orthoester with the molecular formula . Its structure allows it to participate in various chemical reactions, making it a valuable compound in organic chemistry.
Key Applications:
- Solvent in Organic Synthesis: TMOP enhances the solubility of reactants and improves yields in various organic reactions .
- Precursor for Synthesis: It serves as a building block for synthesizing esters and other organic compounds, particularly in the fragrance and flavoring industries .
- Polymer Production: In the polymer industry, TMOP acts as a cross-linking agent, contributing to durable materials with enhanced flexibility and chemical resistance .
- Research in Chemical Biology: It is utilized in biochemical studies, particularly in synthesizing bioactive compounds which aid drug discovery efforts .
Antimicrobial Properties
Recent studies have explored the antimicrobial activity of TMOP against various pathogens. For instance, it has shown effectiveness against strains of Staphylococcus aureus and Candida species. The Minimum Inhibitory Concentration (MIC) values indicate significant antimicrobial potential:
Pathogen | MIC (µg/mL) | MBC (µg/mL) |
---|---|---|
Staphylococcus aureus | 128 | 256 |
Candida albicans | 256 | 512 |
These findings suggest that TMOP may serve as a potential candidate for developing new antimicrobial agents .
Synthesis of Bioactive Compounds
TMOP's role as a reagent in synthesizing bioactive compounds has been documented. For example, it has been used to create prodrugs that exhibit antiviral properties. A notable study involved the synthesis of 2-amino-9-(3-acyloxymethyl-4-alkoxycarbonyloxybut-1-yl)purines using TMOP as a key reagent. These compounds demonstrated effective anti-hepadnaviral responses, reducing viral loads significantly in experimental models .
Case Studies
-
Antimicrobial Activity Against MRSA:
A study evaluated the effectiveness of TMOP against Methicillin-resistant Staphylococcus aureus (MRSA). The results indicated that TMOP inhibited MRSA growth with an MIC value of 128 µg/mL, suggesting its potential utility in treating resistant bacterial infections . -
Synthesis of Antiviral Prodrugs:
In another investigation, TMOP was utilized to synthesize prodrugs of penciclovir. The resulting compounds exhibited high urinary recovery rates and improved antiviral efficacy compared to existing treatments like famciclovir .
Properties
IUPAC Name |
1,1,1-trimethoxypropane | |
---|---|---|
Source | PubChem | |
URL | https://pubchem.ncbi.nlm.nih.gov | |
Description | Data deposited in or computed by PubChem | |
InChI |
InChI=1S/C6H14O3/c1-5-6(7-2,8-3)9-4/h5H2,1-4H3 | |
Source | PubChem | |
URL | https://pubchem.ncbi.nlm.nih.gov | |
Description | Data deposited in or computed by PubChem | |
InChI Key |
ZGMNAIODRDOMEK-UHFFFAOYSA-N | |
Source | PubChem | |
URL | https://pubchem.ncbi.nlm.nih.gov | |
Description | Data deposited in or computed by PubChem | |
Canonical SMILES |
CCC(OC)(OC)OC | |
Source | PubChem | |
URL | https://pubchem.ncbi.nlm.nih.gov | |
Description | Data deposited in or computed by PubChem | |
Molecular Formula |
C6H14O3 | |
Source | PubChem | |
URL | https://pubchem.ncbi.nlm.nih.gov | |
Description | Data deposited in or computed by PubChem | |
DSSTOX Substance ID |
DTXSID00179523 | |
Record name | Propane, 1,1,1-trimethoxy- | |
Source | EPA DSSTox | |
URL | https://comptox.epa.gov/dashboard/DTXSID00179523 | |
Description | DSSTox provides a high quality public chemistry resource for supporting improved predictive toxicology. | |
Molecular Weight |
134.17 g/mol | |
Source | PubChem | |
URL | https://pubchem.ncbi.nlm.nih.gov | |
Description | Data deposited in or computed by PubChem | |
CAS No. |
24823-81-2 | |
Record name | 1,1,1-Trimethoxypropane | |
Source | CAS Common Chemistry | |
URL | https://commonchemistry.cas.org/detail?cas_rn=24823-81-2 | |
Description | CAS Common Chemistry is an open community resource for accessing chemical information. Nearly 500,000 chemical substances from CAS REGISTRY cover areas of community interest, including common and frequently regulated chemicals, and those relevant to high school and undergraduate chemistry classes. This chemical information, curated by our expert scientists, is provided in alignment with our mission as a division of the American Chemical Society. | |
Explanation | The data from CAS Common Chemistry is provided under a CC-BY-NC 4.0 license, unless otherwise stated. | |
Record name | Propane, 1,1,1-trimethoxy- | |
Source | ChemIDplus | |
URL | https://pubchem.ncbi.nlm.nih.gov/substance/?source=chemidplus&sourceid=0024823812 | |
Description | ChemIDplus is a free, web search system that provides access to the structure and nomenclature authority files used for the identification of chemical substances cited in National Library of Medicine (NLM) databases, including the TOXNET system. | |
Record name | Propane, 1,1,1-trimethoxy- | |
Source | EPA DSSTox | |
URL | https://comptox.epa.gov/dashboard/DTXSID00179523 | |
Description | DSSTox provides a high quality public chemistry resource for supporting improved predictive toxicology. | |
Retrosynthesis Analysis
AI-Powered Synthesis Planning: Our tool employs the Template_relevance Pistachio, Template_relevance Bkms_metabolic, Template_relevance Pistachio_ringbreaker, Template_relevance Reaxys, Template_relevance Reaxys_biocatalysis model, leveraging a vast database of chemical reactions to predict feasible synthetic routes.
One-Step Synthesis Focus: Specifically designed for one-step synthesis, it provides concise and direct routes for your target compounds, streamlining the synthesis process.
Accurate Predictions: Utilizing the extensive PISTACHIO, BKMS_METABOLIC, PISTACHIO_RINGBREAKER, REAXYS, REAXYS_BIOCATALYSIS database, our tool offers high-accuracy predictions, reflecting the latest in chemical research and data.
Strategy Settings
Precursor scoring | Relevance Heuristic |
---|---|
Min. plausibility | 0.01 |
Model | Template_relevance |
Template Set | Pistachio/Bkms_metabolic/Pistachio_ringbreaker/Reaxys/Reaxys_biocatalysis |
Top-N result to add to graph | 6 |
Feasible Synthetic Routes
Q1: What is a key analytical challenge in working with Trimethyl orthopropionate, and how has this challenge been addressed?
A1: A key challenge is the accurate and reliable quantification of related substances or impurities present in this compound samples. A recent study [] developed and validated a Gas Chromatography method to address this challenge. This method utilizes a Chemito1000 system equipped with an FID detector and a specific temperature program to separate and quantify this compound and its potential impurities. This validated method provides the necessary sensitivity and accuracy for quality control purposes in various applications.
Q2: What is a novel application of this compound, as highlighted in recent research?
A2: this compound has shown promise as a delayed acid release activator in fracking fluids used in oil and gas extraction []. The compound, incorporated into a tackifying composition coating proppant particles, can be activated under specific downhole conditions. This activation leads to the release of the tackifying agent, improving the performance and placement of proppants in hydraulic fracturing operations.
Disclaimer and Information on In-Vitro Research Products
Please be aware that all articles and product information presented on BenchChem are intended solely for informational purposes. The products available for purchase on BenchChem are specifically designed for in-vitro studies, which are conducted outside of living organisms. In-vitro studies, derived from the Latin term "in glass," involve experiments performed in controlled laboratory settings using cells or tissues. It is important to note that these products are not categorized as medicines or drugs, and they have not received approval from the FDA for the prevention, treatment, or cure of any medical condition, ailment, or disease. We must emphasize that any form of bodily introduction of these products into humans or animals is strictly prohibited by law. It is essential to adhere to these guidelines to ensure compliance with legal and ethical standards in research and experimentation.