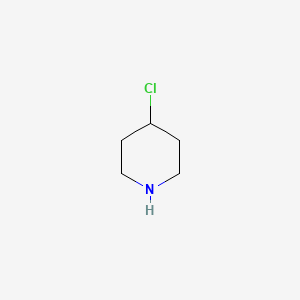
4-Chloropiperidine
Overview
Description
4-Chloropiperidine is an organic compound with the molecular formula C₅H₁₀ClN It is a derivative of piperidine, where a chlorine atom is substituted at the fourth position of the piperidine ring
Scientific Research Applications
4-Chloropiperidine has numerous applications in scientific research:
Biology: It serves as a building block for the synthesis of biologically active molecules.
Medicine: The compound is used in the development of drugs, particularly those targeting the central nervous system.
Industry: It is employed in the production of specialty chemicals and materials.
Safety and Hazards
Mechanism of Action
Target of Action
4-Chloropiperidine is a reactant in the synthesis of indole and benzimidazole piperazines, acting as a histamine H4 receptor antagonist . The primary target of this compound is the histamine H4 receptor, which plays a crucial role in immune response and inflammation.
Mode of Action
The interaction of this compound with its targets involves the formation of an electrophilic aziridinium ion by intramolecular displacement of a chloride, which is then readily attacked by nucleophiles such as the guanine base in DNA .
Biochemical Pathways
This compound affects the histamine signaling pathway. By acting as an antagonist at the histamine H4 receptor, it prevents the receptor from being activated by histamine, thereby inhibiting the downstream effects of histamine signaling .
Pharmacokinetics
It is known that the compound is slightly soluble in chloroform, ethanol, and methanol . This suggests that it may have good bioavailability due to its ability to dissolve in both polar and non-polar solvents.
Result of Action
The molecular and cellular effects of this compound’s action involve the alkylation of DNA, which can lead to DNA damage and cell death . This makes this compound a potential therapeutic agent for conditions such as cancer.
Action Environment
The action of this compound can be influenced by environmental factors such as temperature and pH. For example, the formation of hydrates of this compound is influenced by the temperature, with a significant reduction in the number of hydrates compared with the parent amine at higher temperatures . This suggests that the efficacy and stability of this compound may vary depending on the environmental conditions.
Biochemical Analysis
Biochemical Properties
It is known that piperidine derivatives, such as 4-Chloropiperidine, can interact with various enzymes and proteins . The nature of these interactions is likely dependent on the specific structure and properties of the this compound molecule .
Cellular Effects
Piperidine derivatives have been shown to have various effects on cells, including impacts on cell signaling pathways, gene expression, and cellular metabolism
Molecular Mechanism
It is known that piperidine derivatives can exert their effects at the molecular level through various mechanisms, including binding interactions with biomolecules, enzyme inhibition or activation, and changes in gene expression .
Temporal Effects in Laboratory Settings
It is known that the piperidine ring substitution leads to a significant reduction in the number of hydrates compared with the parent amine . This suggests that this compound may have different stability and degradation properties compared to other piperidine derivatives .
Dosage Effects in Animal Models
It is known that the effects of many drugs can vary with dosage, and this is likely to be the case for this compound as well .
Metabolic Pathways
It is known that piperidine derivatives can be involved in various metabolic pathways
Transport and Distribution
It is known that many drugs can interact with transporters or binding proteins, and can have effects on their localization or accumulation .
Subcellular Localization
It is known that many drugs can be directed to specific compartments or organelles through targeting signals or post-translational modifications .
Preparation Methods
Synthetic Routes and Reaction Conditions
4-Chloropiperidine can be synthesized through several methods. One common approach involves the chlorination of piperidine. This can be achieved by reacting piperidine with thionyl chloride (SOCl₂) under controlled conditions. Another method involves the use of N-chlorosuccinimide (NCS) as a chlorinating agent, which selectively chlorinates the piperidine ring at the fourth position .
Industrial Production Methods
In industrial settings, the production of this compound often involves continuous flow reactions. This method allows for better control over reaction conditions and yields higher purity products. The process typically involves the reaction of piperidine with a chlorinating agent in the presence of a catalyst, followed by purification steps to isolate the desired product .
Chemical Reactions Analysis
Types of Reactions
4-Chloropiperidine undergoes various types of chemical reactions, including:
Substitution Reactions: The chlorine atom in this compound can be substituted by other nucleophiles, such as amines or thiols, to form different derivatives.
Oxidation Reactions: It can be oxidized to form piperidone derivatives.
Reduction Reactions: The compound can be reduced to form piperidine derivatives.
Common Reagents and Conditions
Substitution Reactions: Common reagents include sodium amide (NaNH₂) and thiols. These reactions typically occur under mild conditions.
Oxidation Reactions: Reagents such as potassium permanganate (KMnO₄) or chromium trioxide (CrO₃) are used.
Reduction Reactions: Hydrogen gas (H₂) in the presence of a palladium catalyst (Pd/C) is commonly used for reduction reactions.
Major Products Formed
Substitution Reactions: Various substituted piperidines.
Oxidation Reactions: Piperidone derivatives.
Reduction Reactions: Piperidine derivatives.
Comparison with Similar Compounds
Similar Compounds
4-Chloropyridine: Similar in structure but contains a pyridine ring instead of a piperidine ring.
3-Chloropiperidine: Chlorine atom is at the third position instead of the fourth.
2-Chloropiperidine: Chlorine atom is at the second position.
Uniqueness
4-Chloropiperidine is unique due to its specific substitution pattern, which imparts distinct reactivity and properties compared to its isomers. This makes it particularly useful in the synthesis of certain pharmaceuticals and agrochemicals .
Properties
IUPAC Name |
4-chloropiperidine | |
---|---|---|
Source | PubChem | |
URL | https://pubchem.ncbi.nlm.nih.gov | |
Description | Data deposited in or computed by PubChem | |
InChI |
InChI=1S/C5H10ClN/c6-5-1-3-7-4-2-5/h5,7H,1-4H2 | |
Source | PubChem | |
URL | https://pubchem.ncbi.nlm.nih.gov | |
Description | Data deposited in or computed by PubChem | |
InChI Key |
GBPBXBUHZSOKTH-UHFFFAOYSA-N | |
Source | PubChem | |
URL | https://pubchem.ncbi.nlm.nih.gov | |
Description | Data deposited in or computed by PubChem | |
Canonical SMILES |
C1CNCCC1Cl | |
Source | PubChem | |
URL | https://pubchem.ncbi.nlm.nih.gov | |
Description | Data deposited in or computed by PubChem | |
Molecular Formula |
C5H10ClN | |
Source | PubChem | |
URL | https://pubchem.ncbi.nlm.nih.gov | |
Description | Data deposited in or computed by PubChem | |
DSSTOX Substance ID |
DTXSID70329538 | |
Record name | 4-chloropiperidine | |
Source | EPA DSSTox | |
URL | https://comptox.epa.gov/dashboard/DTXSID70329538 | |
Description | DSSTox provides a high quality public chemistry resource for supporting improved predictive toxicology. | |
Molecular Weight |
119.59 g/mol | |
Source | PubChem | |
URL | https://pubchem.ncbi.nlm.nih.gov | |
Description | Data deposited in or computed by PubChem | |
CAS No. |
5382-18-3 | |
Record name | 4-Chloropiperidine | |
Source | CAS Common Chemistry | |
URL | https://commonchemistry.cas.org/detail?cas_rn=5382-18-3 | |
Description | CAS Common Chemistry is an open community resource for accessing chemical information. Nearly 500,000 chemical substances from CAS REGISTRY cover areas of community interest, including common and frequently regulated chemicals, and those relevant to high school and undergraduate chemistry classes. This chemical information, curated by our expert scientists, is provided in alignment with our mission as a division of the American Chemical Society. | |
Explanation | The data from CAS Common Chemistry is provided under a CC-BY-NC 4.0 license, unless otherwise stated. | |
Record name | 4-chloropiperidine | |
Source | EPA DSSTox | |
URL | https://comptox.epa.gov/dashboard/DTXSID70329538 | |
Description | DSSTox provides a high quality public chemistry resource for supporting improved predictive toxicology. | |
Retrosynthesis Analysis
AI-Powered Synthesis Planning: Our tool employs the Template_relevance Pistachio, Template_relevance Bkms_metabolic, Template_relevance Pistachio_ringbreaker, Template_relevance Reaxys, Template_relevance Reaxys_biocatalysis model, leveraging a vast database of chemical reactions to predict feasible synthetic routes.
One-Step Synthesis Focus: Specifically designed for one-step synthesis, it provides concise and direct routes for your target compounds, streamlining the synthesis process.
Accurate Predictions: Utilizing the extensive PISTACHIO, BKMS_METABOLIC, PISTACHIO_RINGBREAKER, REAXYS, REAXYS_BIOCATALYSIS database, our tool offers high-accuracy predictions, reflecting the latest in chemical research and data.
Strategy Settings
Precursor scoring | Relevance Heuristic |
---|---|
Min. plausibility | 0.01 |
Model | Template_relevance |
Template Set | Pistachio/Bkms_metabolic/Pistachio_ringbreaker/Reaxys/Reaxys_biocatalysis |
Top-N result to add to graph | 6 |
Feasible Synthetic Routes
Q1: What is known about the structural characteristics of 4-chloropiperidine?
A1: this compound is a substituted piperidine derivative. While the provided abstracts don't explicitly state the molecular formula or weight, they do describe its synthesis. [, , ] One study details using this compound hydrochloride as a starting material. [] Another focuses on synthesizing this compound from 4-piperidones or 4-pyridone. [] These studies highlight the importance of the compound's structure in chemical reactions and modifications.
Q2: How does this compound form hydrates, and what is unique about them?
A2: Research indicates that this compound can form both monohydrate and trihydrate structures. [, ] Interestingly, the trihydrate exhibits the same L4(6)5(7)6(8) water-layer motif as the trihydrate of 4-methylpiperidine, despite differences in amine-water hydrogen bond arrangements. [, ] This suggests a potential influence of the piperidine ring on water organization in these hydrates. Additionally, chlorine-chlorine interactions are observed in the this compound hydrate, though their contribution to stability requires further investigation. []
Q3: Have there been any computational studies on this compound and its hydrates?
A3: Yes, periodic DFT-D3 calculations were used to investigate the energetics of this compound trihydrate. [] Results revealed that the energy of water layers was similar to that observed in 4-methylpiperidine trihydrate. [] This computational approach provides valuable insights into the stability and interactions within the hydrate structures.
Q4: Are there any efficient synthetic routes for producing this compound?
A4: Two efficient synthetic routes to 1-tert-butyl-4-chloropiperidine, a derivative of this compound, have been reported. [] One method utilizes thionyl chloride-mediated chlorination with tetrabutylammonium chloride to suppress unwanted side reactions. [] The second, a novel approach, generates the tert-butyl group via the addition of methylmagnesium chloride to a dimethyliminium salt. [] Another study utilizes piperidin-4-one hydrochloride as a raw material to synthesize this compound hydrochloride. [] These findings offer valuable strategies for the preparation of this compound and its derivatives.
Q5: Has the synthesis of this compound from homoallylic amines been explored?
A5: Yes, a recent study explored a novel approach using HCl·DMPU to generate a reactive electrophile from DMSO. [] This electrophile reacts with homoallylic amines, both aromatic and aliphatic, to produce 4-chloropiperidines in good yields. [] This method, utilizing HCl·DMPU as a dual-purpose reagent, presents a potentially more environmentally friendly route for this compound synthesis.
Disclaimer and Information on In-Vitro Research Products
Please be aware that all articles and product information presented on BenchChem are intended solely for informational purposes. The products available for purchase on BenchChem are specifically designed for in-vitro studies, which are conducted outside of living organisms. In-vitro studies, derived from the Latin term "in glass," involve experiments performed in controlled laboratory settings using cells or tissues. It is important to note that these products are not categorized as medicines or drugs, and they have not received approval from the FDA for the prevention, treatment, or cure of any medical condition, ailment, or disease. We must emphasize that any form of bodily introduction of these products into humans or animals is strictly prohibited by law. It is essential to adhere to these guidelines to ensure compliance with legal and ethical standards in research and experimentation.