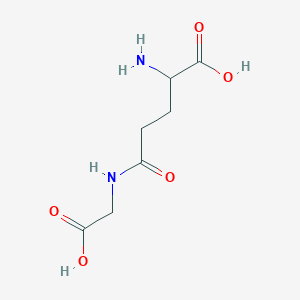
gamma-Glutamylglycine
Overview
Description
Gamma-glutamylglycine (γ-Glu-Gly) is a dipeptide consisting of gamma-linked glutamic acid and glycine. It is classified under amino acids and derivatives, with the molecular formula C₇H₁₂N₂O₅ and a molecular weight of 204.18 g/mol . Structurally, it resembles gamma-aminobutyric acid (GABA), enabling it to act as an excitatory amino acid receptor antagonist . Key roles include:
- Metabolic Regulation: Involved in lipid metabolism and gut microbiota interactions, particularly in the production of GABA precursors .
- Clinical Biomarker: Associated with renal function (e.g., urinary albumin-to-creatinine ratio (UACR) and estimated glomerular filtration rate (eGFR)) and identified as a tier 1 biomarker for chronic kidney disease (CKD) .
- Therapeutic Potential: Modulates epileptic activity by increasing seizure threshold currents in animal models .
Preparation Methods
Enzymatic Synthesis of Gamma-Glutamylglycine
Enzymatic methods dominate gamma-glutamyl peptide synthesis due to their stereospecificity and mild reaction conditions. Two primary pathways have been identified: (1) de novo synthesis via γ-glutamylcysteine ligase (GCL) and glutathione synthetase (GS) homologs, and (2) transpeptidation using γ-glutamyltransferases (GGTs) .
De Novo Synthesis Using Glutathione Biosynthetic Enzymes
The glutathione biosynthetic pathway in S. cerevisiae demonstrates broad substrate flexibility. GCL (encoded by GSH1) catalyzes the ATP-dependent formation of γ-glutamyl dipeptides from glutamate and amino acids, while GS (encoded by GSH2) adds glycine to form tripeptides . For this compound, this pathway could theoretically involve:
-
GCL-mediated synthesis of γ-glutamylglycine from glutamate and glycine.
-
GS-mediated elongation , though this step may be unnecessary for dipeptide formation.
In vitro studies show that GCL from S. cerevisiae accepts glycine as a substrate, albeit with lower efficiency compared to cysteine . Reaction optimization (e.g., pH 8.5, 30°C, 5 mM ATP) enhances yield, but scalability remains limited by enzyme stability and ATP dependency .
Transpeptidation via Gamma-Glutamyltransferases
GGTs transfer the γ-glutamyl moiety from donors like glutathione (GSH) to acceptors such as glycine. The Bacillus subtilis NX-2 GGT has been engineered for industrial applications, achieving 95% purity and high activity (59.6 U/mg protein) . A patented method for γ-glutamylcysteine synthesis illustrates the process:
-
Substrate preparation : S-benzyl-L-cysteine is chemically synthesized.
-
Enzymatic reaction : GGT (1% v/v) catalyzes transpeptidation between L-glutamine and glycine at pH 9.0 and 37°C .
-
Purification : Centrifugation, ammonium sulfate precipitation, and hydrophobic interaction chromatography yield >95% pure product .
For this compound, replacing S-benzyl-L-cysteine with glycine could enable direct synthesis. Recent work with E. coli GGT successfully produced γ-glutamylvalylglycine from glutamine and valylglycine, suggesting adaptability for glycine acceptors .
Microbial Fermentation in Saccharomyces cerevisiae
S. cerevisiae naturally produces γ-glutamyl peptides via intracellular pathways. This compound synthesis could leverage:
-
Alternative GSH degradation : The Dug2p-Dug3p complex transfers γ-glutamyl groups from GSH to dipeptides .
-
Extracellular uptake : Opt1p imports γ-glutamyl peptides, enabling extracellular accumulation .
In a minimal synthetic medium, wild-type yeast produced γ-glutamylvalylglycine (γ-EVG) at 0.2–0.5 mM concentrations . Fermentation parameters influencing yield include:
Parameter | Optimal Condition | Effect on Yield |
---|---|---|
pH | 6.5–7.5 | Maximizes enzyme activity |
Temperature | 30°C | Supports cell viability |
Oxygenation | Aerobic | Enhances GSH synthesis |
Peptide extraction involves heat treatment (70°C for 10 min), centrifugation, and ultrafiltration (10 kDa cutoff) . This method avoids costly chromatography, making it viable for large-scale production.
Chemical Synthesis Approaches
Chemical synthesis of γ-glutamylglycine remains underexplored but offers advantages in scalability. Solid-phase peptide synthesis (SPPS) using Fmoc-protected glutamic acid and glycine is feasible but challenged by the γ-linkage specificity. Key steps include:
-
Protection : Tert-butyl esters for glutamate side chains.
-
Coupling : Dicyclohexylcarbodiimide (DCC) activates the γ-carboxyl group.
-
Deprotection : Trifluoroacetic acid (TFA) cleaves protecting groups.
Yields from SPPS are typically low (<30%) due to steric hindrance and side reactions. Solution-phase synthesis, as described in Patent CN101215588A, uses S-benzyl protection for cysteine , which could be adapted for glycine by omitting benzylation.
Comparative Analysis of Methods
Method | Yield | Cost | Scalability | Purity |
---|---|---|---|---|
Enzymatic (GGT) | High (80–95%) | Moderate | High | >95% |
Microbial Fermentation | Moderate | Low | Moderate | 70–85% |
Chemical Synthesis | Low (20–30%) | High | Low | 90–95% |
Enzymatic methods are preferred for industrial use, while fermentation suits cost-sensitive applications. Chemical synthesis is limited to small-scale research.
Chemical Reactions Analysis
Types of Reactions
H-GAMMA-D-GLU-GLY-OH can undergo various chemical reactions, including:
Oxidation: The compound can be oxidized under specific conditions, leading to the formation of oxidized derivatives.
Reduction: Reduction reactions can convert oxidized forms back to their reduced state.
Substitution: The compound can participate in substitution reactions, where functional groups are replaced by other groups.
Common Reagents and Conditions
Common reagents used in these reactions include oxidizing agents like hydrogen peroxide or potassium permanganate for oxidation, and reducing agents like sodium borohydride for reduction. Substitution reactions may involve nucleophiles or electrophiles depending on the desired transformation .
Major Products Formed
The major products formed from these reactions depend on the specific conditions and reagents used. For example, oxidation may yield oxidized derivatives, while reduction can regenerate the original compound. Substitution reactions can produce various substituted derivatives .
Scientific Research Applications
Biochemical Functions and Mechanisms
Neurotransmitter Modulation
γ-GG has been studied for its role in modulating neurotransmitter systems. It acts as a competitive antagonist at glutamate receptors, influencing synaptic transmission and plasticity. Research indicates that γ-GG can inhibit excitatory neurotransmission, which may have implications for neurological disorders characterized by excessive glutamate signaling .
Metabolic Profiling in Disease
Recent metabolomic studies have identified γ-glutamyl amino acids, including γ-GG, as potential biomarkers for various cancers. In a study analyzing serum metabolites associated with lethal prostate cancer, γ-GG was found to correlate with increased risk, highlighting its potential as a diagnostic marker .
Industrial Applications
Biocatalysis
Gamma-glutamyl transpeptidase (GGT), the enzyme that catalyzes the synthesis of γ-GG, is being explored for its industrial applications in green chemistry. GGT facilitates the production of bioactive peptides and compounds through enzymatic processes that are more sustainable compared to traditional chemical methods . This enzyme's ability to synthesize γ-glutamyl compounds makes it valuable in food technology and pharmaceuticals.
Clinical Applications
Diagnostic Marker for Diseases
GGT is recognized as a diagnostic marker for liver diseases and various cancers. Elevated levels of GGT in serum can indicate pathological conditions, making it a crucial component in clinical diagnostics . The use of γ-glutamyl-[1-13C]glycine in non-invasive imaging techniques has shown promise for monitoring glioblastoma, demonstrating its relevance in cancer diagnostics .
Table 1: Summary of Research Findings on gamma-Glutamylglycine Applications
Mechanism of Action
H-GAMMA-D-GLU-GLY-OH exerts its effects by competitively binding to AMPA receptors, thereby inhibiting the action of glutamate, the primary excitatory neurotransmitter in the central nervous system. This inhibition reduces excitatory synaptic transmission, which can have various physiological effects depending on the context .
Comparison with Similar Compounds
Comparison with Structurally Similar Compounds
Gamma-glutamylglycine belongs to the broader class of gamma-glutamyl dipeptides, which share a gamma-linked glutamic acid but differ in the second amino acid residue. Below is a detailed comparison:
Gamma-Glutamylalanine (γ-Glu-Ala)
- Structure : Glutamic acid + alanine.
- Role : Identified in tuberculosis (TB) research as part of a metabolite panel (with γ-Glu-Gly, glutamine, and pyridoxate) that predicts treatment response (AUC = 0.86) .
- Key Difference : While γ-Glu-Gly is linked to renal and neurological pathways, γ-Glu-Ala is specific to infectious disease monitoring.
Gamma-Glutamylisoleucine (γ-Glu-Ile)
- Structure : Glutamic acid + isoleucine.
- Molecular Formula : C₁₁H₂₀N₂O₅ (MW: 260.29 g/mol) .
- Role : A dipeptide biomarker detected in metabolomic studies but lacks documented receptor antagonist activity .
Gamma-Glutamylleucine (γ-Glu-Leu)
- Structure : Glutamic acid + leucine.
Gamma-D-Glutamylglycine (γ-DGG)
- Structure : Stereoisomer of γ-Glu-Gly with D-configuration.
- Role: A broad-spectrum ionotropic glutamate receptor antagonist used in pharmacological research .
- Key Difference : The D-form exhibits distinct receptor binding properties compared to the L-form (γ-Glu-Gly), impacting its efficacy in modulating excitatory neurotransmission .
Comparison with Larger Gamma-Glutamyl Compounds
Poly-γ-Glutamic Acid (γ-PGA)
- Structure : Polymer of glutamic acid with gamma-linkages.
- Role : Used in biomedical applications (e.g., drug delivery) due to its biodegradability .
- Key Difference: Unlike the dipeptide γ-Glu-Gly, γ-PGA’s polymeric structure enables nanoparticle formation but lacks receptor-specific activity .
Gamma-Glutamylpolyamines
- Structure : Gamma-glutamyl conjugates of polyamines (e.g., spermidine).
- Role : Synthesized by bacteria under stress, facilitating pH homeostasis .
Functional and Clinical Differences
Clinical Relevance
- γ-Glu-Gly : Strong association with CKD (tier 1 biomarker) and UACR reduction (β = -2.444, P < 0.001) .
- γ-Glu-Ala: Limited to TB diagnostics, lacking renal or neurological links .
Structural and Physicochemical Properties
Biological Activity
Gamma-Glutamylglycine (γ-Glu-Gly) is a dipeptide formed from the amino acids glutamate and glycine. It plays significant roles in various biological processes, particularly in the context of cellular metabolism, oxidative stress, and neurogenesis. This article explores the biological activity of γ-Glu-Gly, supported by research findings, case studies, and data tables.
Overview of this compound
This compound is part of the gamma-glutamyl cycle, which is crucial for the synthesis and regulation of glutathione (GSH), a key antioxidant in the body. The compound is involved in various metabolic pathways and has been shown to influence cellular functions related to oxidative stress and inflammation.
1. Role in Neurogenesis
Research indicates that γ-Glu-Gly is involved in neurogenesis through its interaction with specific enzymes. For instance, studies have demonstrated that Botch, a γ-glutamyl cyclotransferase, can deglycinate Notch1 by cleaving γ-Glu-Gly. This process inhibits Notch1 signaling, which is essential for regulating neurogenesis .
2. Antioxidant Activity
As a precursor to glutathione synthesis, γ-Glu-Gly contributes to cellular defense against oxidative stress. Elevated levels of glutathione are associated with reduced oxidative damage, which is critical in various pathological conditions including neurodegenerative diseases .
3. Impact on Cystine Transport
In human pancreatic duct cells, γ-Glu-Gly has been shown to inhibit cystine transport significantly. This inhibition suggests a regulatory role in amino acid uptake mechanisms that are vital for maintaining cellular redox balance and supporting GSH synthesis .
Case Study 1: Neurogenesis Regulation
A study involving embryonic neurogenesis found that the absence of Botch's GGCT activity led to increased Notch1 signaling and impaired neurogenesis. The introduction of γ-Glu-Gly restored normal signaling pathways, highlighting its regulatory role in neural development .
Case Study 2: Oxidative Stress Response
In a cohort study examining oxidative stress markers, participants with higher levels of γ-Glu-Gly showed improved antioxidant defenses. This correlation underscores its potential as a biomarker for assessing oxidative stress-related diseases .
Data Tables
Biological Activity | Effect | Reference |
---|---|---|
Neurogenesis Regulation | Inhibition of Notch1 signaling | |
Antioxidant Activity | Increased GSH levels | |
Cystine Transport | Inhibition by γ-Glu-Gly |
Mechanistic Insights
The mechanisms through which γ-Glu-Gly exerts its biological effects include:
- Modulation of Enzyme Activity : By influencing the activity of γ-glutamyl transferase (GGT) and other enzymes in the gamma-glutamyl cycle.
- Regulation of Cellular Signaling : Through its interactions with receptors involved in calcium signaling and other pathways that affect cell proliferation and survival.
Q & A
Basic Research Questions
Q. How can researchers confirm the structural similarity between gamma-glutamylglycine (γ-Glu-Gly) and GABA?
- Methodological Answer : Structural confirmation requires comparative analysis using nuclear magnetic resonance (NMR) spectroscopy and X-ray crystallography. Computational modeling of γ-Glu-Gly's γ-glutamyl dipeptide structure can highlight its resemblance to GABA, particularly in the positioning of functional groups like carboxyl and amino moieties. Cross-referencing with known GABA receptor binding assays can validate structural mimicry .
Q. What analytical techniques are optimal for detecting γ-Glu-Gly in biological samples?
- Methodological Answer : Liquid chromatography-mass spectrometry (LC-MS) is preferred for high sensitivity and specificity. Enzymatic assays using γ-glutamyl transferase (GGT) can quantify γ-Glu-Gly by measuring its cleavage products. Metabolomic studies, such as those tracking post-exercise metabolite fluctuations, utilize multivariate statistical analysis (e.g., AUC calculations) to identify γ-Glu-Gly's downregulation in physiological stress models .
Q. What is γ-Glu-Gly’s role in lipid metabolism and endogenous signaling pathways?
- Methodological Answer : As a human lipid metabolite, γ-Glu-Gly modulates excitatory amino acid pathways via antagonism. In vitro studies in S. cerevisiae demonstrate its synthesis via GGT, linking it to γ-glutamyl peptide metabolism. In vivo models, such as epilepsy threshold experiments, show its regulatory effects on neuronal excitability through GABA-like mechanisms .
Advanced Research Questions
Q. How should experimental designs be structured to investigate γ-Glu-Gly’s impact on metabotropic glutamate receptors (mGluRs)?
- Methodological Answer : Use selective mGluR agonists/antagonists (e.g., DHPG) in tandem with γ-Glu-Gly to assess competitive binding via radioligand displacement assays. Electrophysiological recordings in hippocampal slices can quantify changes in synaptic plasticity. Integrate phospholipase D (PLD) coupling assays to explore downstream signaling cascades .
Q. How can contradictory data on γ-Glu-Gly’s physiological roles be resolved?
- Methodological Answer : Context-dependent factors (e.g., stress models vs. baseline conditions) must be standardized. For example, ultramarathon studies show γ-Glu-Gly downregulation post-exercise (log2FC=-7.98), suggesting cardio-protective roles, while epilepsy models highlight its excitatory antagonism. Meta-analyses of multi-omics datasets (transcriptomics, metabolomics) can reconcile these disparities .
Q. What methodologies are critical for elucidating γ-Glu-Gly’s dipeptide absorption and bioavailability?
- Methodological Answer : Employ isotopic labeling (e.g., ¹³C/¹⁵N) to track γ-Glu-Gly uptake in intestinal epithelial cell lines. Compare absorption kinetics with amino acids using Ussing chamber assays. Validate in vivo via gastric G-cell activation studies, measuring gastrin secretion rates in response to dipeptide administration .
Q. How can γ-Glu-Gly’s neuroprotective effects be mechanistically dissected in neurodegenerative disease models?
- Methodological Answer : Use transgenic animal models (e.g., Alzheimer’s disease mice) to assess γ-Glu-Gly’s modulation of glutamate excitotoxicity. Combine microdialysis with high-performance liquid chromatography (HPLC) to measure extracellular glutamate levels. Validate via behavioral assays (e.g., Morris water maze) and immunohistochemical analysis of neuronal survival .
Q. Guidance for Data Reporting and Reproducibility
- Experimental Replication : Detailed protocols for γ-Glu-Gly synthesis (e.g., GGT enzymatic activity in S. cerevisiae) must include substrate concentrations, pH, and temperature controls .
- Statistical Rigor : Use AUC (area under the curve) metrics for metabolomic data, as seen in ultramarathon studies, to ensure robustness .
- Ethical Compliance : For in vivo studies, adhere to ARRIVE guidelines for animal research, particularly in epilepsy and neurodegenerative models .
Properties
IUPAC Name |
2-amino-5-(carboxymethylamino)-5-oxopentanoic acid | |
---|---|---|
Source | PubChem | |
URL | https://pubchem.ncbi.nlm.nih.gov | |
Description | Data deposited in or computed by PubChem | |
InChI |
InChI=1S/C7H12N2O5/c8-4(7(13)14)1-2-5(10)9-3-6(11)12/h4H,1-3,8H2,(H,9,10)(H,11,12)(H,13,14) | |
Source | PubChem | |
URL | https://pubchem.ncbi.nlm.nih.gov | |
Description | Data deposited in or computed by PubChem | |
InChI Key |
ACIJGUBIMXQCMF-UHFFFAOYSA-N | |
Source | PubChem | |
URL | https://pubchem.ncbi.nlm.nih.gov | |
Description | Data deposited in or computed by PubChem | |
Canonical SMILES |
C(CC(=O)NCC(=O)O)C(C(=O)O)N | |
Source | PubChem | |
URL | https://pubchem.ncbi.nlm.nih.gov | |
Description | Data deposited in or computed by PubChem | |
Molecular Formula |
C7H12N2O5 | |
Source | PubChem | |
URL | https://pubchem.ncbi.nlm.nih.gov | |
Description | Data deposited in or computed by PubChem | |
Molecular Weight |
204.18 g/mol | |
Source | PubChem | |
URL | https://pubchem.ncbi.nlm.nih.gov | |
Description | Data deposited in or computed by PubChem | |
CAS No. |
1948-29-4 | |
Record name | gamma-Glutamylglycine | |
Source | ChemIDplus | |
URL | https://pubchem.ncbi.nlm.nih.gov/substance/?source=chemidplus&sourceid=0001948294 | |
Description | ChemIDplus is a free, web search system that provides access to the structure and nomenclature authority files used for the identification of chemical substances cited in National Library of Medicine (NLM) databases, including the TOXNET system. | |
Record name | Glycine, N-L-.gamma.-glutamyl- | |
Source | DTP/NCI | |
URL | https://dtp.cancer.gov/dtpstandard/servlet/dwindex?searchtype=NSC&outputformat=html&searchlist=334201 | |
Description | The NCI Development Therapeutics Program (DTP) provides services and resources to the academic and private-sector research communities worldwide to facilitate the discovery and development of new cancer therapeutic agents. | |
Explanation | Unless otherwise indicated, all text within NCI products is free of copyright and may be reused without our permission. Credit the National Cancer Institute as the source. | |
Retrosynthesis Analysis
AI-Powered Synthesis Planning: Our tool employs the Template_relevance Pistachio, Template_relevance Bkms_metabolic, Template_relevance Pistachio_ringbreaker, Template_relevance Reaxys, Template_relevance Reaxys_biocatalysis model, leveraging a vast database of chemical reactions to predict feasible synthetic routes.
One-Step Synthesis Focus: Specifically designed for one-step synthesis, it provides concise and direct routes for your target compounds, streamlining the synthesis process.
Accurate Predictions: Utilizing the extensive PISTACHIO, BKMS_METABOLIC, PISTACHIO_RINGBREAKER, REAXYS, REAXYS_BIOCATALYSIS database, our tool offers high-accuracy predictions, reflecting the latest in chemical research and data.
Strategy Settings
Precursor scoring | Relevance Heuristic |
---|---|
Min. plausibility | 0.01 |
Model | Template_relevance |
Template Set | Pistachio/Bkms_metabolic/Pistachio_ringbreaker/Reaxys/Reaxys_biocatalysis |
Top-N result to add to graph | 6 |
Feasible Synthetic Routes
Disclaimer and Information on In-Vitro Research Products
Please be aware that all articles and product information presented on BenchChem are intended solely for informational purposes. The products available for purchase on BenchChem are specifically designed for in-vitro studies, which are conducted outside of living organisms. In-vitro studies, derived from the Latin term "in glass," involve experiments performed in controlled laboratory settings using cells or tissues. It is important to note that these products are not categorized as medicines or drugs, and they have not received approval from the FDA for the prevention, treatment, or cure of any medical condition, ailment, or disease. We must emphasize that any form of bodily introduction of these products into humans or animals is strictly prohibited by law. It is essential to adhere to these guidelines to ensure compliance with legal and ethical standards in research and experimentation.