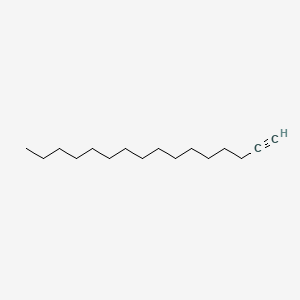
1-Hexadecyne
Overview
Description
C₁₆H₃₀ . It is a long-chain alkyne, characterized by the presence of a triple bond between the first and second carbon atoms in the chain. This compound is a member of the alkynes family, which are hydrocarbons containing at least one carbon-carbon triple bond. This compound is a colorless liquid at room temperature and is used in various chemical synthesis processes .
Mechanism of Action
is a long-chain hydrocarbon with the molecular formula C16H30 . It is one of many isomers of hexadecene .
In terms of safety, it is recommended to avoid dust formation, breathing mist, gas or vapours, and contacting with skin and eye when handling 1-Hexadecyne . Use of personal protective equipment and ensuring adequate ventilation are advised .
Biochemical Analysis
Biochemical Properties
1-Hexadecyne plays a significant role in biochemical reactions, particularly in the context of lipid metabolism. It interacts with various enzymes and proteins involved in these pathways. For instance, this compound can act as a substrate for certain desaturase enzymes, which introduce double bonds into fatty acid chains. This interaction is crucial for the modification of lipid molecules, affecting their fluidity and function within cellular membranes .
Cellular Effects
The effects of this compound on cellular processes are diverse. It has been observed to influence cell signaling pathways, gene expression, and cellular metabolism. In particular, this compound can modulate the activity of key signaling molecules, such as protein kinases, which play a role in various cellular responses. Additionally, it can affect the expression of genes involved in lipid metabolism, leading to changes in the composition and function of cellular membranes .
Molecular Mechanism
At the molecular level, this compound exerts its effects through specific binding interactions with biomolecules. It can inhibit or activate enzymes by binding to their active sites, altering their catalytic activity. For example, this compound has been shown to inhibit the activity of certain lipases, enzymes that break down lipids, by binding to their active sites and preventing substrate access. This inhibition can lead to an accumulation of lipid molecules within cells .
Temporal Effects in Laboratory Settings
In laboratory settings, the effects of this compound can change over time due to its stability and degradation. Studies have shown that this compound is relatively stable under standard laboratory conditions, but it can degrade over extended periods, leading to a decrease in its biological activity. Long-term exposure to this compound in in vitro or in vivo studies has been associated with alterations in cellular function, including changes in lipid metabolism and membrane composition .
Dosage Effects in Animal Models
The effects of this compound vary with different dosages in animal models. At low doses, this compound can modulate lipid metabolism without causing significant toxicity. At high doses, it can induce toxic effects, such as liver damage and disruptions in lipid homeostasis. These adverse effects are likely due to the accumulation of unmetabolized this compound and its interference with essential biochemical pathways .
Metabolic Pathways
This compound is involved in several metabolic pathways, particularly those related to lipid metabolism. It interacts with enzymes such as desaturases and elongases, which modify the structure of fatty acids. These interactions can affect the metabolic flux of lipid molecules, leading to changes in the levels of various metabolites. Additionally, this compound can influence the activity of enzymes involved in the synthesis and degradation of lipids, further impacting metabolic pathways .
Transport and Distribution
Within cells and tissues, this compound is transported and distributed through interactions with specific transporters and binding proteins. These interactions facilitate its localization to cellular membranes, where it can exert its effects on lipid metabolism. The distribution of this compound within cells is influenced by its hydrophobic nature, which allows it to integrate into lipid bilayers and accumulate in membrane compartments .
Subcellular Localization
The subcellular localization of this compound is primarily within cellular membranes, where it can affect membrane fluidity and function. It is directed to specific compartments or organelles through targeting signals and post-translational modifications. For example, this compound can be targeted to the endoplasmic reticulum, where it interacts with enzymes involved in lipid synthesis and modification .
Preparation Methods
Synthetic Routes and Reaction Conditions: 1-Hexadecyne can be synthesized through several methods. One common approach involves the alkylation of acetylene with a long-chain alkyl halide. This reaction typically requires a strong base, such as sodium amide, to deprotonate the acetylene and generate the acetylide anion, which then reacts with the alkyl halide to form the desired alkyne.
Industrial Production Methods: In industrial settings, this compound can be produced through the catalytic dehydrogenation of hexadecane. This process involves the removal of hydrogen atoms from hexadecane in the presence of a catalyst, such as platinum or palladium, at elevated temperatures. The resulting product is then purified through distillation to obtain pure this compound .
Chemical Reactions Analysis
Types of Reactions: 1-Hexadecyne undergoes various chemical reactions, including:
Oxidation: this compound can be oxidized to form hexadecanoic acid using oxidizing agents such as potassium permanganate or ozone.
Reduction: The triple bond in this compound can be reduced to form hexadecane using hydrogen gas in the presence of a metal catalyst like palladium on carbon.
Substitution: this compound can undergo nucleophilic substitution reactions where the triple bond is attacked by nucleophiles, leading to the formation of substituted alkynes.
Common Reagents and Conditions:
Oxidation: Potassium permanganate, ozone, and other strong oxidizing agents.
Reduction: Hydrogen gas, palladium on carbon, and other metal catalysts.
Substitution: Nucleophiles such as halides, amines, and alcohols.
Major Products Formed:
Oxidation: Hexadecanoic acid.
Reduction: Hexadecane.
Substitution: Various substituted alkynes depending on the nucleophile used.
Scientific Research Applications
1-Hexadecyne has several applications in scientific research, including:
Chemistry: Used as a building block in organic synthesis to create more complex molecules.
Biology: Employed in the study of lipid metabolism and the synthesis of bioactive lipids.
Medicine: Investigated for its potential use in drug delivery systems and as a precursor for the synthesis of pharmaceuticals.
Industry: Utilized in the production of specialty chemicals, surfactants, and lubricants.
Comparison with Similar Compounds
1-Hexadecene: An alkene with a double bond between the first and second carbon atoms.
Hexadecane: A saturated hydrocarbon with no double or triple bonds.
1-Octadecyne: An alkyne with a similar structure but with two additional carbon atoms.
Comparison: 1-Hexadecyne is unique due to the presence of the carbon-carbon triple bond, which imparts distinct reactivity compared to alkenes and alkanes. The triple bond makes this compound more reactive in addition reactions compared to 1-Hexadecene, which has a double bond. Compared to hexadecane, which is relatively inert, this compound can participate in a wider range of chemical reactions. 1-Octadecyne, while similar in structure, has a longer carbon chain, which can affect its physical properties and reactivity .
Properties
IUPAC Name |
hexadec-1-yne | |
---|---|---|
Source | PubChem | |
URL | https://pubchem.ncbi.nlm.nih.gov | |
Description | Data deposited in or computed by PubChem | |
InChI |
InChI=1S/C16H30/c1-3-5-7-9-11-13-15-16-14-12-10-8-6-4-2/h1H,4-16H2,2H3 | |
Source | PubChem | |
URL | https://pubchem.ncbi.nlm.nih.gov | |
Description | Data deposited in or computed by PubChem | |
InChI Key |
UCIDYSLOTJMRAM-UHFFFAOYSA-N | |
Source | PubChem | |
URL | https://pubchem.ncbi.nlm.nih.gov | |
Description | Data deposited in or computed by PubChem | |
Canonical SMILES |
CCCCCCCCCCCCCCC#C | |
Source | PubChem | |
URL | https://pubchem.ncbi.nlm.nih.gov | |
Description | Data deposited in or computed by PubChem | |
Molecular Formula |
C16H30 | |
Source | PubChem | |
URL | https://pubchem.ncbi.nlm.nih.gov | |
Description | Data deposited in or computed by PubChem | |
DSSTOX Substance ID |
DTXSID2060880 | |
Record name | 1-Hexadecyne | |
Source | EPA DSSTox | |
URL | https://comptox.epa.gov/dashboard/DTXSID2060880 | |
Description | DSSTox provides a high quality public chemistry resource for supporting improved predictive toxicology. | |
Molecular Weight |
222.41 g/mol | |
Source | PubChem | |
URL | https://pubchem.ncbi.nlm.nih.gov | |
Description | Data deposited in or computed by PubChem | |
CAS No. |
629-74-3 | |
Record name | 1-Hexadecyne | |
Source | CAS Common Chemistry | |
URL | https://commonchemistry.cas.org/detail?cas_rn=629-74-3 | |
Description | CAS Common Chemistry is an open community resource for accessing chemical information. Nearly 500,000 chemical substances from CAS REGISTRY cover areas of community interest, including common and frequently regulated chemicals, and those relevant to high school and undergraduate chemistry classes. This chemical information, curated by our expert scientists, is provided in alignment with our mission as a division of the American Chemical Society. | |
Explanation | The data from CAS Common Chemistry is provided under a CC-BY-NC 4.0 license, unless otherwise stated. | |
Record name | 1-Hexadecyne | |
Source | ChemIDplus | |
URL | https://pubchem.ncbi.nlm.nih.gov/substance/?source=chemidplus&sourceid=0000629743 | |
Description | ChemIDplus is a free, web search system that provides access to the structure and nomenclature authority files used for the identification of chemical substances cited in National Library of Medicine (NLM) databases, including the TOXNET system. | |
Record name | 1-Hexadecyne | |
Source | EPA Chemicals under the TSCA | |
URL | https://www.epa.gov/chemicals-under-tsca | |
Description | EPA Chemicals under the Toxic Substances Control Act (TSCA) collection contains information on chemicals and their regulations under TSCA, including non-confidential content from the TSCA Chemical Substance Inventory and Chemical Data Reporting. | |
Record name | 1-Hexadecyne | |
Source | EPA DSSTox | |
URL | https://comptox.epa.gov/dashboard/DTXSID2060880 | |
Description | DSSTox provides a high quality public chemistry resource for supporting improved predictive toxicology. | |
Record name | Hexadec-1-yne | |
Source | European Chemicals Agency (ECHA) | |
URL | https://echa.europa.eu/substance-information/-/substanceinfo/100.010.098 | |
Description | The European Chemicals Agency (ECHA) is an agency of the European Union which is the driving force among regulatory authorities in implementing the EU's groundbreaking chemicals legislation for the benefit of human health and the environment as well as for innovation and competitiveness. | |
Explanation | Use of the information, documents and data from the ECHA website is subject to the terms and conditions of this Legal Notice, and subject to other binding limitations provided for under applicable law, the information, documents and data made available on the ECHA website may be reproduced, distributed and/or used, totally or in part, for non-commercial purposes provided that ECHA is acknowledged as the source: "Source: European Chemicals Agency, http://echa.europa.eu/". Such acknowledgement must be included in each copy of the material. ECHA permits and encourages organisations and individuals to create links to the ECHA website under the following cumulative conditions: Links can only be made to webpages that provide a link to the Legal Notice page. | |
Retrosynthesis Analysis
AI-Powered Synthesis Planning: Our tool employs the Template_relevance Pistachio, Template_relevance Bkms_metabolic, Template_relevance Pistachio_ringbreaker, Template_relevance Reaxys, Template_relevance Reaxys_biocatalysis model, leveraging a vast database of chemical reactions to predict feasible synthetic routes.
One-Step Synthesis Focus: Specifically designed for one-step synthesis, it provides concise and direct routes for your target compounds, streamlining the synthesis process.
Accurate Predictions: Utilizing the extensive PISTACHIO, BKMS_METABOLIC, PISTACHIO_RINGBREAKER, REAXYS, REAXYS_BIOCATALYSIS database, our tool offers high-accuracy predictions, reflecting the latest in chemical research and data.
Strategy Settings
Precursor scoring | Relevance Heuristic |
---|---|
Min. plausibility | 0.01 |
Model | Template_relevance |
Template Set | Pistachio/Bkms_metabolic/Pistachio_ringbreaker/Reaxys/Reaxys_biocatalysis |
Top-N result to add to graph | 6 |
Feasible Synthetic Routes
Q1: How does 1-hexadecyne interact with hydrogen-terminated silicon surfaces, and what are the advantages of this interaction?
A1: this compound forms self-assembled monolayers (SAMs) on hydrogen-terminated silicon (H-Si) surfaces through a process called hydrosilylation. This reaction involves the addition of the Si-H bond across the carbon-carbon triple bond of the alkyne, resulting in the formation of a stable Si-C bond. [, ] This direct covalent attachment leads to robust and well-defined organic monolayers. Compared to alkenes like 1-hexadecene, this compound exhibits faster monolayer formation and improved surface coverage on H-Si(111) due to the higher reactivity of its terminal alkyne group. []
Q2: Can you elaborate on the structural features of this compound, including its molecular formula, weight, and spectroscopic data?
A2: this compound has the molecular formula C16H30 and a molecular weight of 222.41 g/mol. [, , ] Spectroscopically, the terminal alkyne group is characterized by a distinct C≡C stretching band around 2100 cm-1 in infrared spectroscopy. [] Nuclear magnetic resonance (NMR) spectroscopy would reveal characteristic signals for the terminal alkyne proton and the aliphatic chain.
Q3: How does the chain length of alkynes, including this compound, affect the electronic properties of ruthenium nanoparticle films?
A3: Research suggests that the chain length of alkyne ligands significantly influences the electronic conductivity of ruthenium nanoparticle films. [] Shorter alkyne chains (1-hexyne to 1-decyne) result in a lower coupling coefficient (β) compared to longer chains (1-dodecyne to this compound). This difference is attributed to the relative contributions of the conjugated metal-ligand interfacial bonding interactions and the saturated aliphatic backbones. Longer chains provide a more significant contribution from the conjugated system, facilitating interparticle charge transfer and enhancing conductivity. []
Q4: What is the significance of identifying this compound in the essential oil of various plant species?
A4: The presence of this compound, alongside other compounds, has been identified in the essential oils of several plant species like Cinnamomum kanehirae [] and Potentilla arbuscula var. veitchii. [] While the specific role of this compound within these plants is not explicitly discussed in the provided research, its presence contributes to the overall chemical profile of the essential oils, which often play roles in plant defense mechanisms, pollination, and communication.
Q5: How is gas chromatography-mass spectrometry (GC-MS) employed in the study of this compound?
A5: GC-MS is a powerful analytical technique used to separate, identify, and quantify the components of complex mixtures. [, , , ] In the context of this compound, GC-MS enables its detection and quantification in various samples like plant extracts [, , , ] and essential oils. [, ] The technique relies on the different retention times of compounds within a chromatographic column and their unique mass fragmentation patterns for identification.
Q6: What are the potential applications of this compound in material science and beyond?
A6: this compound's ability to form stable and well-defined SAMs on silicon surfaces makes it promising for developing microelectronic devices and sensors. [] The controlled functionalization of surfaces with this compound can tailor properties like wettability, adhesion, and electrical conductivity. Furthermore, its presence in plant extracts and its identification through GC-MS suggest potential applications in areas such as natural product chemistry, fragrance development, and traditional medicine.
Disclaimer and Information on In-Vitro Research Products
Please be aware that all articles and product information presented on BenchChem are intended solely for informational purposes. The products available for purchase on BenchChem are specifically designed for in-vitro studies, which are conducted outside of living organisms. In-vitro studies, derived from the Latin term "in glass," involve experiments performed in controlled laboratory settings using cells or tissues. It is important to note that these products are not categorized as medicines or drugs, and they have not received approval from the FDA for the prevention, treatment, or cure of any medical condition, ailment, or disease. We must emphasize that any form of bodily introduction of these products into humans or animals is strictly prohibited by law. It is essential to adhere to these guidelines to ensure compliance with legal and ethical standards in research and experimentation.