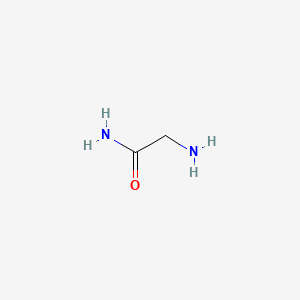
Glycinamide
Overview
Description
Mechanism of Action
Target of Action
Glycinamide, also known as 2-Aminoacetamide, is a small molecule that primarily targets the enzyme Neutrophil collagenase . This enzyme plays a crucial role in the degradation of the extracellular matrix, which is a key process in various physiological and pathological conditions .
Mode of Action
It is known that this compound can act as a ligand for transition metals , which might influence its interaction with its target.
Biochemical Pathways
This compound is involved in the de novo purine biosynthesis pathway . It is used in the synthesis of glycineamide ribonucleotide, an intermediate in this pathway . The de novo purine biosynthesis pathway is crucial for the production of purine nucleotides, which are essential building blocks for DNA and RNA.
Pharmacokinetics
It is known that this compound is a water-soluble compound , which suggests that it could have good bioavailability. More research is needed to fully understand the pharmacokinetic properties of this compound.
Action Environment
The action, efficacy, and stability of this compound could be influenced by various environmental factors. For instance, the pH of the environment could potentially affect the stability of this compound, as it is known that the hydrochloride salt of this compound has a pKa near the physiological pH . More research is needed to fully understand how environmental factors influence the action of this compound.
Biochemical Analysis
Biochemical Properties
Glycinamide plays a significant role in biochemical reactions, particularly in the synthesis of glycineamide ribonucleotide. It interacts with several enzymes and proteins, including this compound ribonucleotide synthetase (PurD), which catalyzes the conversion of phosphoribosylamine, glycine, and ATP to this compound ribonucleotide, ADP, and inorganic phosphate . Additionally, this compound acts as a ligand for transition metals, forming complexes that can influence various biochemical pathways .
Cellular Effects
This compound has been shown to influence various cellular processes. It facilitates nanocomplex formation and works synergistically with bone morphogenetic protein 2 to promote osteoblast differentiation and bone regeneration . In human dermal fibroblasts, this compound, in combination with ascorbic acid, enhances collagen production and wound healing . These effects suggest that this compound plays a crucial role in cell signaling pathways, gene expression, and cellular metabolism.
Molecular Mechanism
At the molecular level, this compound exerts its effects through several mechanisms. It readily forms nanocomplexes, facilitating cellular uptake through strong electrostatic interactions . This compound also interacts with enzymes such as this compound ribonucleotide synthetase, influencing the synthesis of glycineamide ribonucleotide . These interactions can lead to changes in gene expression and enzyme activity, contributing to its overall biochemical effects.
Temporal Effects in Laboratory Settings
In laboratory settings, the effects of this compound can change over time. This compound hydrochloride, a derivative of this compound, has a pKa near the physiological pH, making it useful in cell culture work . Its stability and degradation over time can influence its long-term effects on cellular function. Studies have shown that this compound can maintain its activity in various experimental conditions, contributing to its effectiveness in biochemical research .
Dosage Effects in Animal Models
The effects of this compound vary with different dosages in animal models. In studies involving bone regeneration, this compound, in combination with bone morphogenetic protein 2, demonstrated enhanced bone volume and mature bone formation at specific dosages . High doses of this compound may lead to toxic or adverse effects, highlighting the importance of dosage optimization in experimental settings.
Metabolic Pathways
This compound is involved in several metabolic pathways, including the de novo purine biosynthesis pathway. It is a key reagent in the synthesis of glycineamide ribonucleotide, an intermediate in this pathway . This compound interacts with enzymes such as this compound ribonucleotide synthetase, influencing metabolic flux and metabolite levels in cells .
Transport and Distribution
Within cells and tissues, this compound is transported and distributed through various mechanisms. It interacts with transporters and binding proteins that facilitate its localization and accumulation in specific cellular compartments . These interactions can influence the overall distribution and effectiveness of this compound in biochemical processes.
Subcellular Localization
This compound’s subcellular localization plays a crucial role in its activity and function. Studies have shown that this compound ribonucleotide synthetase, an enzyme that interacts with this compound, displays a typical chloroplast location pattern in rice protoplasts . This localization is essential for the enzyme’s role in purine nucleotide biosynthesis and highlights the importance of subcellular targeting in this compound’s biochemical effects.
Preparation Methods
Synthetic Routes and Reaction Conditions: Glycinamide can be synthesized by treating the amino acid ester of glycine with ammonia . This method is advantageous due to its moderate reaction conditions, high yield, and minimal by-products .
Industrial Production Methods: Industrial production of this compound often employs the hydrolysis of glycinonitrile hydrochloride due to its efficiency and scalability . This method involves dissolving glycinonitrile hydrochloride in isopropanol, heating to 50-70°C, and introducing dried hydrogen chloride gas until saturation .
Chemical Reactions Analysis
Types of Reactions: Glycinamide undergoes various chemical reactions, including:
Hydrolysis: this compound can be hydrolyzed to form glycine.
Complexation: It acts as a ligand for transition metals, forming complexes with metals such as cobalt, nickel, and copper.
Common Reagents and Conditions:
Hydrolysis: Typically involves water and an acid or base catalyst.
Complexation: Involves transition metal salts and this compound under controlled conditions.
Major Products:
Hydrolysis: Glycine.
Complexation: Metal-glycinamide complexes.
Scientific Research Applications
Glycinamide has diverse applications in scientific research:
Comparison with Similar Compounds
Glycine: The parent amino acid of glycinamide.
Glycine Methyl Ester: Another derivative of glycine with different properties.
N-Acetyl Glycine: A derivative of glycine with acetylation.
Uniqueness: this compound is unique due to its dual role as a ligand for transition metals and its biological activity in promoting collagen production and osteoblast differentiation . Unlike other glycine derivatives, this compound has shown significant potential in both biochemical and industrial applications .
Properties
IUPAC Name |
2-aminoacetamide | |
---|---|---|
Source | PubChem | |
URL | https://pubchem.ncbi.nlm.nih.gov | |
Description | Data deposited in or computed by PubChem | |
InChI |
InChI=1S/C2H6N2O/c3-1-2(4)5/h1,3H2,(H2,4,5) | |
Source | PubChem | |
URL | https://pubchem.ncbi.nlm.nih.gov | |
Description | Data deposited in or computed by PubChem | |
InChI Key |
BEBCJVAWIBVWNZ-UHFFFAOYSA-N | |
Source | PubChem | |
URL | https://pubchem.ncbi.nlm.nih.gov | |
Description | Data deposited in or computed by PubChem | |
Canonical SMILES |
C(C(=O)N)N | |
Source | PubChem | |
URL | https://pubchem.ncbi.nlm.nih.gov | |
Description | Data deposited in or computed by PubChem | |
Molecular Formula |
C2H6N2O | |
Source | PubChem | |
URL | https://pubchem.ncbi.nlm.nih.gov | |
Description | Data deposited in or computed by PubChem | |
Related CAS |
1668-10-6 (hydrochloride), 598-41-4 (Parent) | |
Record name | Glycine amide | |
Source | ChemIDplus | |
URL | https://pubchem.ncbi.nlm.nih.gov/substance/?source=chemidplus&sourceid=0000598414 | |
Description | ChemIDplus is a free, web search system that provides access to the structure and nomenclature authority files used for the identification of chemical substances cited in National Library of Medicine (NLM) databases, including the TOXNET system. | |
Record name | Glycinamide hydrochloride | |
Source | ChemIDplus | |
URL | https://pubchem.ncbi.nlm.nih.gov/substance/?source=chemidplus&sourceid=0001668106 | |
Description | ChemIDplus is a free, web search system that provides access to the structure and nomenclature authority files used for the identification of chemical substances cited in National Library of Medicine (NLM) databases, including the TOXNET system. | |
DSSTOX Substance ID |
DTXSID1060508 | |
Record name | Acetamide, 2-amino- | |
Source | EPA DSSTox | |
URL | https://comptox.epa.gov/dashboard/DTXSID1060508 | |
Description | DSSTox provides a high quality public chemistry resource for supporting improved predictive toxicology. | |
Molecular Weight |
74.08 g/mol | |
Source | PubChem | |
URL | https://pubchem.ncbi.nlm.nih.gov | |
Description | Data deposited in or computed by PubChem | |
CAS No. |
598-41-4, 1668-10-6 | |
Record name | Glycinamide | |
Source | CAS Common Chemistry | |
URL | https://commonchemistry.cas.org/detail?cas_rn=598-41-4 | |
Description | CAS Common Chemistry is an open community resource for accessing chemical information. Nearly 500,000 chemical substances from CAS REGISTRY cover areas of community interest, including common and frequently regulated chemicals, and those relevant to high school and undergraduate chemistry classes. This chemical information, curated by our expert scientists, is provided in alignment with our mission as a division of the American Chemical Society. | |
Explanation | The data from CAS Common Chemistry is provided under a CC-BY-NC 4.0 license, unless otherwise stated. | |
Record name | Glycine amide | |
Source | ChemIDplus | |
URL | https://pubchem.ncbi.nlm.nih.gov/substance/?source=chemidplus&sourceid=0000598414 | |
Description | ChemIDplus is a free, web search system that provides access to the structure and nomenclature authority files used for the identification of chemical substances cited in National Library of Medicine (NLM) databases, including the TOXNET system. | |
Record name | Glycinamide hydrochloride | |
Source | ChemIDplus | |
URL | https://pubchem.ncbi.nlm.nih.gov/substance/?source=chemidplus&sourceid=0001668106 | |
Description | ChemIDplus is a free, web search system that provides access to the structure and nomenclature authority files used for the identification of chemical substances cited in National Library of Medicine (NLM) databases, including the TOXNET system. | |
Record name | Glycinamide | |
Source | DrugBank | |
URL | https://www.drugbank.ca/drugs/DB03636 | |
Description | The DrugBank database is a unique bioinformatics and cheminformatics resource that combines detailed drug (i.e. chemical, pharmacological and pharmaceutical) data with comprehensive drug target (i.e. sequence, structure, and pathway) information. | |
Explanation | Creative Common's Attribution-NonCommercial 4.0 International License (http://creativecommons.org/licenses/by-nc/4.0/legalcode) | |
Record name | Acetamide, 2-amino- | |
Source | EPA Chemicals under the TSCA | |
URL | https://www.epa.gov/chemicals-under-tsca | |
Description | EPA Chemicals under the Toxic Substances Control Act (TSCA) collection contains information on chemicals and their regulations under TSCA, including non-confidential content from the TSCA Chemical Substance Inventory and Chemical Data Reporting. | |
Record name | Acetamide, 2-amino- | |
Source | EPA DSSTox | |
URL | https://comptox.epa.gov/dashboard/DTXSID1060508 | |
Description | DSSTox provides a high quality public chemistry resource for supporting improved predictive toxicology. | |
Record name | 2-aminoacetamide | |
Source | European Chemicals Agency (ECHA) | |
URL | https://echa.europa.eu/substance-information/-/substanceinfo/100.009.031 | |
Description | The European Chemicals Agency (ECHA) is an agency of the European Union which is the driving force among regulatory authorities in implementing the EU's groundbreaking chemicals legislation for the benefit of human health and the environment as well as for innovation and competitiveness. | |
Explanation | Use of the information, documents and data from the ECHA website is subject to the terms and conditions of this Legal Notice, and subject to other binding limitations provided for under applicable law, the information, documents and data made available on the ECHA website may be reproduced, distributed and/or used, totally or in part, for non-commercial purposes provided that ECHA is acknowledged as the source: "Source: European Chemicals Agency, http://echa.europa.eu/". Such acknowledgement must be included in each copy of the material. ECHA permits and encourages organisations and individuals to create links to the ECHA website under the following cumulative conditions: Links can only be made to webpages that provide a link to the Legal Notice page. | |
Record name | GLYCINAMIDE | |
Source | FDA Global Substance Registration System (GSRS) | |
URL | https://gsrs.ncats.nih.gov/ginas/app/beta/substances/4JDT453NWO | |
Description | The FDA Global Substance Registration System (GSRS) enables the efficient and accurate exchange of information on what substances are in regulated products. Instead of relying on names, which vary across regulatory domains, countries, and regions, the GSRS knowledge base makes it possible for substances to be defined by standardized, scientific descriptions. | |
Explanation | Unless otherwise noted, the contents of the FDA website (www.fda.gov), both text and graphics, are not copyrighted. They are in the public domain and may be republished, reprinted and otherwise used freely by anyone without the need to obtain permission from FDA. Credit to the U.S. Food and Drug Administration as the source is appreciated but not required. | |
Record name | glycinamide | |
Source | Human Metabolome Database (HMDB) | |
URL | http://www.hmdb.ca/metabolites/HMDB0062472 | |
Description | The Human Metabolome Database (HMDB) is a freely available electronic database containing detailed information about small molecule metabolites found in the human body. | |
Explanation | HMDB is offered to the public as a freely available resource. Use and re-distribution of the data, in whole or in part, for commercial purposes requires explicit permission of the authors and explicit acknowledgment of the source material (HMDB) and the original publication (see the HMDB citing page). We ask that users who download significant portions of the database cite the HMDB paper in any resulting publications. | |
Synthesis routes and methods
Procedure details
Retrosynthesis Analysis
AI-Powered Synthesis Planning: Our tool employs the Template_relevance Pistachio, Template_relevance Bkms_metabolic, Template_relevance Pistachio_ringbreaker, Template_relevance Reaxys, Template_relevance Reaxys_biocatalysis model, leveraging a vast database of chemical reactions to predict feasible synthetic routes.
One-Step Synthesis Focus: Specifically designed for one-step synthesis, it provides concise and direct routes for your target compounds, streamlining the synthesis process.
Accurate Predictions: Utilizing the extensive PISTACHIO, BKMS_METABOLIC, PISTACHIO_RINGBREAKER, REAXYS, REAXYS_BIOCATALYSIS database, our tool offers high-accuracy predictions, reflecting the latest in chemical research and data.
Strategy Settings
Precursor scoring | Relevance Heuristic |
---|---|
Min. plausibility | 0.01 |
Model | Template_relevance |
Template Set | Pistachio/Bkms_metabolic/Pistachio_ringbreaker/Reaxys/Reaxys_biocatalysis |
Top-N result to add to graph | 6 |
Feasible Synthetic Routes
Q1: What is glycinamide's role in purine biosynthesis?
A1: this compound is a key intermediate in the de novo purine biosynthesis pathway. It is converted to N-formylthis compound ribonucleotide by the enzyme this compound ribonucleotide transformylase (GART), using 10-formyltetrahydrofolate as a cofactor [, ]. This pathway is crucial for the synthesis of purine nucleotides, essential building blocks of DNA and RNA.
Q2: How does the human this compound ribonucleotide transformylase (GART) interact with this compound ribonucleotide?
A2: The human GART domain binds to this compound ribonucleotide in an ordered-sequential manner, with the folate substrate binding first []. The enzyme exhibits flexibility in its active site, as evidenced by a disordered loop in the apo structure that becomes ordered upon binding to this compound ribonucleotide and a folate inhibitor [].
Q3: Can you elaborate on the structural features of this compound ribonucleotide that are crucial for enzymatic activity with human GART?
A3: Studies utilizing various analogs of this compound ribonucleotide have shed light on the structural requirements for enzymatic activity with human GART. The enzyme demonstrates acceptance for the sarcosyl analog, carbocyclic this compound ribonucleotide, and specific phosphonate derivatives, albeit with varying affinities []. This suggests a degree of flexibility in substrate recognition, while certain modifications, particularly at the phosphate group, can significantly impact binding.
Q4: How does the inhibition of GART affect purine biosynthesis?
A4: Inhibiting GART disrupts the conversion of this compound ribonucleotide to N-formylthis compound ribonucleotide, leading to a decrease in formylthis compound ribonucleotide levels []. This depletion ultimately hinders the de novo purine biosynthesis pathway, impacting the production of purine nucleotides.
Q5: What is the molecular formula and weight of this compound?
A5: The molecular formula of this compound is C2H6N2O, and its molecular weight is 74.08 g/mol.
Q6: How have computational studies been used to investigate the conformational behavior of this compound?
A6: Density Functional Theory (DFT) calculations have been instrumental in understanding the conformational behavior of this compound [, , ]. These studies explored the impact of solvation on various this compound conformers, revealing that the peptide bond strengthens while the C=O bond weakens in aqueous solutions. Additionally, DFT was used to examine the multiwater-assisted proton transfers within this compound, providing insights into the thermodynamic and kinetic aspects of these processes [].
Q7: Have there been any studies using computational methods to understand the interaction of this compound with other molecules?
A7: Yes, DFT calculations have been employed to study the interaction of this compound with sodium chloride []. These studies suggest that this compound conformers exhibit a preference for interaction with the {111} surface of sodium chloride, potentially influencing the crystal habit of sodium chloride.
Q8: How do modifications to the this compound structure affect its activity as an adenosine kinase inhibitor?
A8: Replacing the hydrophobic C4-phenylamino substituent in diaryltubercidin analogues with a more hydrophilic this compound group led to improved water solubility while maintaining adenosine kinase inhibition potency []. Further modifications to the this compound moiety, base, and sugar were explored to optimize this balance.
Q9: How do structural modifications to this compound ribonucleotide analogs impact their interaction with this compound ribonucleotide synthetase?
A9: Various modifications to this compound ribonucleotide have been explored to understand their impact on this compound ribonucleotide synthetase activity []. Replacing the glycine side chain with larger groups, modifying the ribose ring with cyclopentane or cyclopentene derivatives, and substituting the phosphate group with phosphonates generally resulted in analogs that could still act as substrates, but with altered binding affinities and reaction rates.
Q10: How is milacemide, a this compound derivative, metabolized in the body?
A10: Milacemide is primarily metabolized in the brain by monoamine oxidase B (MAO-B) to this compound, which is further converted to glycine [, ]. This metabolism is supported by studies showing that pretreatment with the MAO-B inhibitor l-deprenyl significantly reduces this compound formation and increases milacemide accumulation in the cerebrospinal fluid [].
Q11: What are the pharmacokinetic properties of valproyl this compound isomers?
A11: Two new isomers of valproyl this compound, valnoctyl this compound (VCGD) and diisopropylacetyl this compound (DIGD), have shown promising anticonvulsant activity. Following intravenous administration in dogs, VCGD exhibited a clearance of 3.8±1.1 L/h, a volume of distribution of 15±2 L, and a half-life of 1.9±0.3 h []. DIGD had a clearance of 10±0.8 L/h, a volume of distribution of 19±3 L, and a half-life of 1.6±0.2 h []. Both compounds were partially metabolized to their corresponding glycine analogues.
Q12: What is the evidence for the anti-melanogenic activity of this compound hydrochloride?
A12: A double-blinded clinical trial demonstrated that topical application of this compound hydrochloride significantly reduced melanin index and increased skin lightness in human subjects compared to a control group []. These findings suggest that this compound hydrochloride holds potential for managing skin hyperpigmentation.
Q13: What are the potential applications of the synergistic effects observed between this compound and ascorbic acid?
A13: A study revealed a synergistic effect between this compound and ascorbic acid in promoting collagen production and wound healing in human dermal fibroblasts []. This combination showed potential for enhancing collagen synthesis and accelerating wound closure, suggesting promising applications in wound healing and tissue regeneration.
Q14: Has milacemide's anticonvulsant activity been investigated in animal models?
A14: Yes, milacemide has demonstrated dose-dependent anticonvulsant activity in various animal models of epilepsy []. It is suggested that its conversion to glycine, an inhibitory neurotransmitter, in the brain contributes to this effect.
Q15: Can you elaborate on the analytical techniques used to assess the bioequivalence of amtolmetin guacil formulations?
A16: A bioequivalence study employed HPLC with UV detection to determine the levels of amtolmetin guacil and its active metabolites, tolmetin sodium and tolmetin this compound, in human plasma []. This method allowed for the quantification of these compounds, enabling the comparison of pharmacokinetic parameters between different formulations.
Q16: What strategies have been explored to enhance the water solubility of adenosine kinase inhibitors?
A17: Adenosine kinase inhibitors like 4-(phenylamino)-5-phenyl-7-(5-deoxy-β-D-ribofuranosyl)pyrrolo[2,3-d]pyrimidine, while potent, suffer from poor water solubility due to the stacking of aryl rings []. Replacing the hydrophobic C4-phenylamino substituent with a more hydrophilic this compound group was found to significantly improve water solubility while preserving inhibitory activity against adenosine kinase [].
Disclaimer and Information on In-Vitro Research Products
Please be aware that all articles and product information presented on BenchChem are intended solely for informational purposes. The products available for purchase on BenchChem are specifically designed for in-vitro studies, which are conducted outside of living organisms. In-vitro studies, derived from the Latin term "in glass," involve experiments performed in controlled laboratory settings using cells or tissues. It is important to note that these products are not categorized as medicines or drugs, and they have not received approval from the FDA for the prevention, treatment, or cure of any medical condition, ailment, or disease. We must emphasize that any form of bodily introduction of these products into humans or animals is strictly prohibited by law. It is essential to adhere to these guidelines to ensure compliance with legal and ethical standards in research and experimentation.