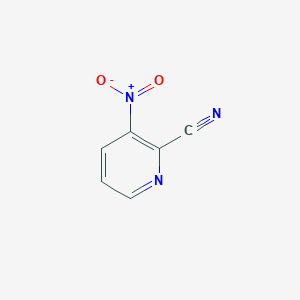
2-Cyano-3-nitropyridine
Overview
Description
2-Cyano-3-nitropyridine (CAS: 51315-07-2) is a pyridine derivative with the molecular formula C₆H₃N₃O₂ and a molecular weight of 149.107 g/mol . It features a cyano group at position 2 and a nitro group at position 3, creating a highly electron-deficient aromatic ring. This compound is a critical intermediate in organic synthesis, particularly in pharmaceuticals and agrochemicals, due to its dual electron-withdrawing groups (cyano and nitro), which enhance reactivity in nucleophilic and electrophilic substitutions . Key physical properties include a density of ~1.4 g/cm³, boiling point of 340.3±27.0 °C, and flash point of 159.6±23.7 °C .
Preparation Methods
Nitration of 2-Cyanopyridine
The most direct and commonly reported method for preparing 2-Cyano-3-nitropyridine involves the nitration of 2-cyanopyridine. This approach utilizes electrophilic aromatic substitution to introduce the nitro group at the 3-position adjacent to the cyano substituent.
-
- Dinitrogen pentoxide (N₂O₅) in organic solvents is used to generate the active nitrating species, proceeding via an N-nitropyridinium ion intermediate.
- Alternatively, a nitrating mixture of concentrated nitric acid (HNO₃) and sulfuric acid (H₂SO₄) can be employed, with sulfuric acid acting as a catalyst to enhance nitration efficiency.
-
- Typically conducted under controlled temperature to avoid over-nitration or decomposition.
- The reaction medium is often an organic solvent compatible with nitrating agents and pyridine derivatives.
-
- Formation of an electrophilic nitronium ion (NO₂⁺) which attacks the pyridine ring at the 3-position relative to the cyano group.
- Rearrangement and stabilization yield this compound.
-
- Large-scale nitration processes optimize reagent ratios, temperature, and reaction time to maximize yield and purity.
- Continuous flow reactors and advanced separation techniques are employed for safety and efficiency.
Parameter | Typical Value/Condition | Notes |
---|---|---|
Nitrating Agent | N₂O₅ or HNO₃/H₂SO₄ mixture | Choice depends on scale and availability |
Solvent | Organic solvents compatible with nitration | Ensures solubility and reaction control |
Temperature | Controlled, often 0–30°C | Prevents side reactions |
Reaction Time | Variable, optimized for complete nitration | Monitored by TLC or other analytical methods |
Yield | High, optimized in industrial processes | Purity critical for downstream applications |
Alternative Synthetic Routes and Precursors
While direct nitration of 2-cyanopyridine is the most straightforward method, other synthetic strategies have been explored, often involving halogenated or amino-substituted pyridine intermediates.
Halogenation and Amination Routes:
- Starting from halogenated pyridine derivatives such as 4-chloro-2-aminopyridine, nitration can be performed to introduce the nitro group.
- Subsequent diazotization and hydrolysis steps convert amino groups to hydroxy or cyano functionalities.
-
- Use of phosphorous oxychloride (POCl₃) in the presence of phase transfer catalysts to prepare dichloronitropyridines.
- Diazotization with sodium nitrite and hydrochloric acid at low temperatures (0–5°C) followed by controlled hydrolysis at elevated temperatures (60–80°C).
- Extraction and purification steps involving dichloromethane and drying agents to isolate intermediates.
-
- Formation of impurities such as dipyridine byproducts.
- Use of hazardous reagents like sulfur dioxide gas.
- Costly reagents (e.g., cesium acetate) and solvents (e.g., acetonitrile).
- Lengthy multi-step processes reducing industrial viability.
-
- Novel processes focus on stable, cost-effective reagents.
- Reduction in the number of synthetic steps.
- Industrial-friendly conditions with minimized impurities.
Detailed Reaction Sequence (Example from Patent Literature)
Step | Reaction Description | Conditions & Reagents | Outcome/Product |
---|---|---|---|
1 | Preparation of 4-chloro-2-aminopyridine | Reaction of 2,4-dihydroxy-3-nitropyridine with POCl₃ and benzyltriethyl ammonium chloride in acetonitrile | 4-chloro-2-aminopyridine |
2 | Nitration of 4-chloro-2-aminopyridine | Nitrating mixture of HNO₃ and H₂SO₄ at 25–30°C | 4-chloro-2-amino-3-nitropyridine |
3 | Diazotization and hydrolysis | Sodium nitrite and HCl at 0–5°C, then heated to 60–80°C | 4-chloro-3-nitropyridin-2-ol |
4 | Conversion to 2-chloro-3-nitropyridin-4-ol | Reaction with sodium acetate in DMF at 120–125°C | 2-chloro-3-nitropyridin-4-ol |
This sequence illustrates a multi-step approach to nitropyridine derivatives, which can be adapted for cyano-substituted analogs with suitable modifications.
Industrial Production Insights
-
- Use of continuous flow reactors to improve heat and mass transfer.
- Careful control of exothermic nitration reactions to maintain safety.
- Selection of solvents and reagents to reduce environmental impact and cost.
-
- Extraction with organic solvents such as dichloromethane.
- Drying over anhydrous sodium sulfate.
- Concentration under reduced pressure to isolate solid products.
- Recrystallization or washing with solvents like toluene or ethyl acetate to enhance purity.
Summary Table of Preparation Methods
Method | Reagents & Conditions | Advantages | Limitations |
---|---|---|---|
Nitration of 2-cyanopyridine | N₂O₅ or HNO₃/H₂SO₄; controlled temperature | Direct, high yield, industrially viable | Requires careful control of conditions |
Halogenation + Amination route | POCl₃, phase transfer catalyst, nitration, diazotization | Allows functional group manipulation | Multi-step, costly reagents |
Diazotization and hydrolysis | NaNO₂, HCl, heating | Converts amino to hydroxy or cyano groups | Requires low temperature control |
Sodium acetate substitution | Sodium acetate in DMF, heating | Efficient substitution at 4-position | Requires handling of high-boiling solvents |
Chemical Reactions Analysis
Types of Reactions
2-Cyano-3-nitropyridine undergoes various chemical reactions, including:
Nucleophilic Substitution: The nitro group at the third position makes the compound susceptible to nucleophilic substitution reactions. Common nucleophiles include amines and thiols.
Reduction: The nitro group can be reduced to an amino group (-NH2) using reducing agents such as hydrogen gas (H2) in the presence of a catalyst like palladium on carbon (Pd/C).
Oxidation: The cyano group can be oxidized to a carboxylic acid group (-COOH) using strong oxidizing agents like potassium permanganate (KMnO4).
Common Reagents and Conditions
Nucleophilic Substitution: Reagents such as sodium methoxide (NaOCH3) or potassium tert-butoxide (KOtBu) in polar aprotic solvents like dimethyl sulfoxide (DMSO) are commonly used.
Reduction: Catalytic hydrogenation using Pd/C or chemical reduction using iron powder (Fe) and hydrochloric acid (HCl).
Oxidation: Potassium permanganate (KMnO4) in aqueous or alkaline conditions.
Major Products Formed
Nucleophilic Substitution: Substituted pyridines with various functional groups depending on the nucleophile used.
Reduction: 2-Cyano-3-aminopyridine.
Oxidation: 2-Carboxy-3-nitropyridine.
Scientific Research Applications
Applications in Organic Synthesis
2-Cyano-3-nitropyridine serves as a key building block in the synthesis of various organic compounds. Its applications include:
Synthesis of Pyridone Derivatives
The compound is used to synthesize 3-cyano-2-pyridones, which exhibit insecticidal properties. A study demonstrated that these derivatives showed significant biological activity against pests, indicating potential agricultural applications .
Functionalization of Aromatic Compounds
The compound acts as a precursor for the functionalization of aromatic compounds through nucleophilic substitution reactions. This property allows for the introduction of diverse functional groups, facilitating the creation of more complex molecular architectures .
Research has indicated that this compound and its derivatives possess notable biological activities:
Insecticidal Properties
Recent studies have shown that derivatives of this compound exhibit insecticidal properties, making them candidates for developing new agrochemicals. For instance, certain synthesized 3-cyano-2-pyridones demonstrated effective pest control capabilities with low lethal concentration values (LC50) .
Antimicrobial Activity
Some derivatives have also been evaluated for their antimicrobial properties, showcasing effectiveness against various bacterial strains. This aspect opens avenues for their use in pharmaceutical formulations targeting infections .
Case Studies and Research Findings
Several studies have documented the applications and efficacy of this compound:
Mechanism of Action
The mechanism of action of 2-Cyano-3-nitropyridine is largely dependent on its chemical reactivity. The nitro group is a strong electron-withdrawing group, which makes the pyridine ring more susceptible to nucleophilic attack. The cyano group also contributes to the compound’s reactivity by stabilizing intermediates formed during chemical reactions. These properties make this compound a versatile intermediate in various chemical transformations.
Comparison with Similar Compounds
Structural and Functional Group Variations
The following table summarizes key structural and functional differences between 2-cyano-3-nitropyridine and its analogs:
Crystallographic and Physical Properties
- This compound: Limited crystallographic data, but linear cyano group likely leads to planar molecular packing. High boiling point indicates strong intermolecular forces .
2-Chloro-3-Nitropyridine :
2-Chloro-5-Methyl-3-Nitropyridine :
- Methyl group induces steric effects, reducing crystal symmetry. C–H⋯O bonds stabilize the lattice .
Biological Activity
2-Cyano-3-nitropyridine is a compound of significant interest in medicinal chemistry and biochemistry due to its diverse biological activities. This article explores its mechanisms of action, cellular effects, and potential applications, supported by relevant data tables and case studies.
Compound Overview
This compound, with the chemical formula CHNO, features a cyano group at the 2-position and a nitro group at the 3-position of the pyridine ring. It is primarily used as a precursor in organic synthesis and exhibits various biological activities that warrant further exploration.
The biological activity of this compound is largely attributed to its ability to interact with specific enzymes and proteins, influencing cellular processes. It is known to undergo nucleophilic substitution reactions, particularly with tetrabutylammonium fluoride (TBAF), resulting in the formation of 2-cyano-3-fluoropyridine. This transformation is crucial as it may alter the compound's reactivity and biological profile.
Cellular Effects
Cellular Interaction:
this compound has been shown to affect various cellular signaling pathways. Its interaction with biomolecules can lead to changes in gene expression and cellular metabolism. For instance, studies indicate that it can influence enzyme activity through competitive inhibition or activation mechanisms.
Toxicity and Dosage Effects:
Research has demonstrated that the compound's effects vary significantly with dosage. Higher concentrations may induce cytotoxicity, while lower doses can promote cell viability. A study on similar nitropyridine derivatives indicated that varying dosages resulted in different levels of enzyme inhibition .
Table 1: Summary of Biological Activities
Biological Activity | Observations/Findings |
---|---|
Enzyme Inhibition | Inhibits specific enzymes involved in metabolic pathways |
Cytotoxicity | Induces cell death at high concentrations |
Gene Expression Modulation | Alters gene expression profiles in treated cells |
Interaction with Transporters | Affects transport mechanisms within cellular environments |
Case Studies
-
Delayed-Type Hypersensitivity (DTH) Model:
In a study assessing the effects of this compound on DTH responses in mice, groups were sensitized with methylated bovine serum albumin (MBSA). The compound was administered orally before antigenic challenges, leading to observable changes in paw edema responses. The results indicated that varying doses influenced the severity of edema, suggesting a dose-dependent effect on immune response modulation . -
In Vitro Cytotoxicity Assays:
Cytotoxicity assays conducted on human cancer cell lines demonstrated that this compound exhibited significant inhibitory effects against several cancer types, including breast and cervical cancer cells. The IC values ranged from 5 μM to 15 μM, indicating potent bioactivity .
Metabolic Pathways
The compound participates in various metabolic pathways, influencing enzyme activity and metabolite levels. Its ability to form derivatives through nucleophilic substitution reactions suggests potential interactions with key metabolic enzymes.
Q & A
Basic Research Questions
Q. What are the recommended synthetic routes for 2-Cyano-3-nitropyridine, and how can its purity be validated?
- Methodological Answer : Synthesis typically involves nitration of pyridine derivatives or substitution reactions. For example, nitration of 2-cyanopyridine under controlled conditions (e.g., HNO₃/H₂SO₄ at 0–5°C) may yield the nitro derivative. Post-synthesis, purity can be validated via HPLC (using C18 columns and acetonitrile/water mobile phases) or GC-MS with electron ionization. Structural confirmation requires ¹H/¹³C NMR (to identify cyano and nitro group positions) and FT-IR (to confirm nitrile stretching bands ~2200 cm⁻¹ and nitro group vibrations ~1500–1350 cm⁻¹). Cross-validate with X-ray crystallography if single crystals are obtainable .
Q. What safety protocols are critical when handling this compound in laboratory settings?
- Methodological Answer : Follow OSHA/EN standards:
- PPE : Wear nitrile gloves, chemical-resistant lab coats, and ANSI-approved goggles to prevent skin/eye contact .
- Respiratory Protection : Use NIOSH-approved N95 respirators for routine handling; in case of spills or thermal decomposition (e.g., releasing NOₓ or HCN), employ a SCBA (self-contained breathing apparatus) .
- Ventilation : Conduct reactions in fume hoods with >100 fpm airflow. Store in airtight containers away from reductants or combustibles .
Advanced Research Questions
Q. How can density-functional theory (DFT) elucidate the electronic structure and reactivity of this compound?
- Methodological Answer : Use hybrid functionals like B3LYP (combining exact exchange and gradient corrections) to model electron distribution and frontier molecular orbitals (HOMO/LUMO). Basis sets such as 6-311++G(d,p) improve accuracy for nitro and cyano groups. Software tools like Gaussian or ORCA can optimize geometries, while cclib parses output files for post-processing (e.g., electrostatic potential maps or charge transfer analysis) . Validate computational results against experimental UV-Vis spectra (λmax for nitroaromatic transitions) .
Q. What strategies resolve contradictions between experimental and computational data for nitro-group reactivity in this compound?
- Methodological Answer :
- Parameter Calibration : Re-evaluate DFT functionals (e.g., test M06-2X for non-covalent interactions) or solvent models (PCM vs. SMD) .
- Experimental Reproducibility : Ensure reaction conditions (temperature, solvent purity) are consistent. Use isotopic labeling (¹⁵N in nitro groups) to track reaction pathways via MS/MS .
- Multi-Method Validation : Compare kinetics (e.g., Arrhenius plots from HPLC data) with transition-state theory calculations .
Q. How can mechanistic studies differentiate between electrophilic and nucleophilic pathways in reactions involving this compound?
- Methodological Answer :
- Kinetic Isotope Effects (KIE) : Replace reactive hydrogens with deuterium to assess bond-breaking steps in nucleophilic attacks .
- Computational Transition States : Identify intermediates using intrinsic reaction coordinate (IRC) analysis in DFT. Compare activation energies for competing pathways .
- Substituent Effects : Synthesize analogs (e.g., 2-Cyano-5-nitropyridine) and compare reaction rates via stopped-flow spectroscopy .
Q. What analytical methods optimize the detection of trace impurities in this compound samples?
- Methodological Answer :
- LC-MS/MS : Use reverse-phase columns (e.g., Zorbax Eclipse Plus) with 0.1% formic acid in mobile phases for high sensitivity. Monitor for common byproducts (e.g., de-nitrated or hydrolyzed derivatives) .
- Headspace GC : Detect volatile impurities (e.g., residual solvents) with flame ionization detectors. Calibrate using EPA Method 8270 for nitroaromatics .
- ICP-OES : Screen for heavy metal catalysts (e.g., Pd, Cu) at ppb levels .
Q. Data Analysis and Interpretation
Q. How should researchers design experiments to assess the environmental persistence of this compound?
- Methodological Answer :
- Hydrolysis Studies : Conduct pH-dependent degradation experiments (pH 3–9) at 25°C and 40°C. Analyze products via HPLC-DAD and compare half-lives to QSAR predictions .
- Photolysis : Exclude samples to UV light (λ=254 nm) and monitor degradation with LC-TOF-MS for accurate mass identification of photoproducts .
- Microbial Degradation : Use OECD 301B ready biodegradability tests with activated sludge; quantify residual compound via SPE-LC/MS .
Q. What statistical approaches are suitable for analyzing dose-response relationships in toxicological studies of this compound?
- Methodological Answer :
- Nonlinear Regression : Fit data to Hill or log-logistic models (e.g., using R’s drc package) to estimate EC₅₀ values .
- ANOVA with Post Hoc Tests : Compare toxicity across concentrations; apply Tukey’s HSD for pairwise comparisons. Validate normality with Shapiro-Wilk tests .
- Bayesian Inference : Use Markov chain Monte Carlo (MCMC) in Stan or PyMC3 to model uncertainty in low-dose extrapolation .
Q. Tables for Key Data
Table 1. Computational Parameters for DFT Studies
Functional | Basis Set | Software | Key Outputs | Validation Method |
---|---|---|---|---|
B3LYP | 6-311++G(d,p) | Gaussian | HOMO/LUMO, Electrostatic Potentials | UV-Vis, X-ray |
M06-2X | def2-TZVP | ORCA | Reaction Barriers | Kinetic Data |
Table 2. Analytical Methods for Impurity Detection
Technique | Target Impurities | Detection Limit | Reference Standard |
---|---|---|---|
LC-MS/MS | De-nitrated derivatives | 0.1 ppm | EPA 8270 |
Headspace GC-FID | Residual solvents (e.g., DCM) | 10 ppb | USP <467> |
Properties
IUPAC Name |
3-nitropyridine-2-carbonitrile | |
---|---|---|
Source | PubChem | |
URL | https://pubchem.ncbi.nlm.nih.gov | |
Description | Data deposited in or computed by PubChem | |
InChI |
InChI=1S/C6H3N3O2/c7-4-5-6(9(10)11)2-1-3-8-5/h1-3H | |
Source | PubChem | |
URL | https://pubchem.ncbi.nlm.nih.gov | |
Description | Data deposited in or computed by PubChem | |
InChI Key |
OLXPGXMJZQJQHT-UHFFFAOYSA-N | |
Source | PubChem | |
URL | https://pubchem.ncbi.nlm.nih.gov | |
Description | Data deposited in or computed by PubChem | |
Canonical SMILES |
C1=CC(=C(N=C1)C#N)[N+](=O)[O-] | |
Source | PubChem | |
URL | https://pubchem.ncbi.nlm.nih.gov | |
Description | Data deposited in or computed by PubChem | |
Molecular Formula |
C6H3N3O2 | |
Source | PubChem | |
URL | https://pubchem.ncbi.nlm.nih.gov | |
Description | Data deposited in or computed by PubChem | |
DSSTOX Substance ID |
DTXSID30340926 | |
Record name | 2-Cyano-3-nitropyridine | |
Source | EPA DSSTox | |
URL | https://comptox.epa.gov/dashboard/DTXSID30340926 | |
Description | DSSTox provides a high quality public chemistry resource for supporting improved predictive toxicology. | |
Molecular Weight |
149.11 g/mol | |
Source | PubChem | |
URL | https://pubchem.ncbi.nlm.nih.gov | |
Description | Data deposited in or computed by PubChem | |
CAS No. |
51315-07-2 | |
Record name | 2-Cyano-3-nitropyridine | |
Source | EPA DSSTox | |
URL | https://comptox.epa.gov/dashboard/DTXSID30340926 | |
Description | DSSTox provides a high quality public chemistry resource for supporting improved predictive toxicology. | |
Synthesis routes and methods
Procedure details
Retrosynthesis Analysis
AI-Powered Synthesis Planning: Our tool employs the Template_relevance Pistachio, Template_relevance Bkms_metabolic, Template_relevance Pistachio_ringbreaker, Template_relevance Reaxys, Template_relevance Reaxys_biocatalysis model, leveraging a vast database of chemical reactions to predict feasible synthetic routes.
One-Step Synthesis Focus: Specifically designed for one-step synthesis, it provides concise and direct routes for your target compounds, streamlining the synthesis process.
Accurate Predictions: Utilizing the extensive PISTACHIO, BKMS_METABOLIC, PISTACHIO_RINGBREAKER, REAXYS, REAXYS_BIOCATALYSIS database, our tool offers high-accuracy predictions, reflecting the latest in chemical research and data.
Strategy Settings
Precursor scoring | Relevance Heuristic |
---|---|
Min. plausibility | 0.01 |
Model | Template_relevance |
Template Set | Pistachio/Bkms_metabolic/Pistachio_ringbreaker/Reaxys/Reaxys_biocatalysis |
Top-N result to add to graph | 6 |
Feasible Synthetic Routes
Disclaimer and Information on In-Vitro Research Products
Please be aware that all articles and product information presented on BenchChem are intended solely for informational purposes. The products available for purchase on BenchChem are specifically designed for in-vitro studies, which are conducted outside of living organisms. In-vitro studies, derived from the Latin term "in glass," involve experiments performed in controlled laboratory settings using cells or tissues. It is important to note that these products are not categorized as medicines or drugs, and they have not received approval from the FDA for the prevention, treatment, or cure of any medical condition, ailment, or disease. We must emphasize that any form of bodily introduction of these products into humans or animals is strictly prohibited by law. It is essential to adhere to these guidelines to ensure compliance with legal and ethical standards in research and experimentation.