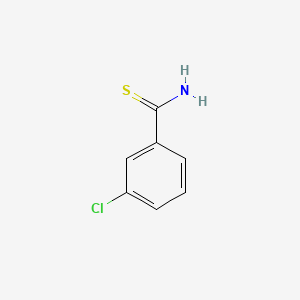
3-Chlorothiobenzamide
Overview
Description
3-Chlorothiobenzamide: is an organic compound with the molecular formula C7H6ClNS and a molecular weight of 171.65 g/mol . It is a derivative of benzamide where the amide group is substituted with a thiocarbonyl group and a chlorine atom is attached to the benzene ring at the third position. This compound is primarily used in research settings and has various applications in chemical synthesis and biological studies.
Mechanism of Action
Action Environment
Environmental factors can influence the action, efficacy, and stability of 3-Chlorothiobenzamide . Factors such as temperature and pH can affect the structure of the compound and its ability to interact with its targets. Additionally, the presence of other molecules in the environment can also influence the compound’s action.
Biochemical Analysis
Biochemical Properties
3-Chlorothiobenzamide plays a significant role in various biochemical reactions. It interacts with several enzymes, proteins, and other biomolecules. For instance, it has been observed to interact with proteases, which are enzymes that break down proteins into smaller peptides or amino acids . The nature of these interactions often involves the inhibition of protease activity, thereby affecting protein degradation processes . Additionally, this compound can bind to specific protein receptors, altering their conformation and function .
Cellular Effects
The effects of this compound on cellular processes are profound. It influences cell function by modulating cell signaling pathways, gene expression, and cellular metabolism . For example, this compound has been shown to inhibit certain signaling pathways, leading to reduced cell proliferation and increased apoptosis in cancer cells . It also affects gene expression by altering the transcriptional activity of specific genes involved in cell cycle regulation and apoptosis . Furthermore, this compound impacts cellular metabolism by inhibiting key metabolic enzymes, leading to altered metabolic fluxes .
Molecular Mechanism
At the molecular level, this compound exerts its effects through several mechanisms. It binds to specific biomolecules, such as enzymes and receptors, leading to their inhibition or activation . For instance, this compound inhibits proteases by binding to their active sites, preventing substrate access and subsequent catalysis . This inhibition can result in the accumulation of undegraded proteins and peptides within cells . Additionally, this compound can modulate gene expression by interacting with transcription factors, thereby influencing the transcriptional activity of target genes .
Temporal Effects in Laboratory Settings
The effects of this compound in laboratory settings can vary over time. Studies have shown that the compound is relatively stable under standard laboratory conditions, with minimal degradation observed over extended periods . Its long-term effects on cellular function can be significant. Prolonged exposure to this compound can lead to sustained inhibition of protease activity, resulting in the accumulation of undegraded proteins and peptides . This can ultimately affect cellular homeostasis and function .
Dosage Effects in Animal Models
The effects of this compound vary with different dosages in animal models. At low doses, the compound has been shown to inhibit protease activity without causing significant toxicity . At higher doses, this compound can induce toxic effects, including liver and kidney damage . Threshold effects have also been observed, where a certain dosage is required to achieve significant inhibition of protease activity . These findings highlight the importance of careful dosage optimization in experimental settings .
Metabolic Pathways
This compound is involved in several metabolic pathways. It interacts with enzymes such as cytochrome P450s, which are involved in the metabolism of various xenobiotics . These interactions can lead to the formation of metabolites that may have different biological activities compared to the parent compound . Additionally, this compound can affect metabolic fluxes by inhibiting key metabolic enzymes, leading to altered levels of metabolites within cells .
Transport and Distribution
The transport and distribution of this compound within cells and tissues are mediated by specific transporters and binding proteins . For instance, the compound can be transported across cell membranes by organic anion transporters, facilitating its uptake into cells . Once inside the cell, this compound can bind to intracellular proteins, influencing its localization and accumulation . These interactions can affect the compound’s bioavailability and efficacy .
Subcellular Localization
This compound exhibits specific subcellular localization patterns. It has been observed to localize primarily in the cytoplasm, where it interacts with various cytoplasmic proteins and enzymes . Additionally, this compound can be targeted to specific organelles, such as the endoplasmic reticulum and mitochondria, through post-translational modifications and targeting signals . These localization patterns can influence the compound’s activity and function within cells .
Preparation Methods
Synthetic Routes and Reaction Conditions: 3-Chlorothiobenzamide can be synthesized through several methods. One common method involves the reaction of 3-chlorobenzoyl chloride with ammonium thiocyanate in the presence of a base such as pyridine . The reaction typically proceeds under reflux conditions, and the product is isolated by crystallization.
Industrial Production Methods: While specific industrial production methods for this compound are not widely documented, the synthesis generally follows similar principles as laboratory methods but on a larger scale. This involves the use of industrial-grade reagents and optimized reaction conditions to ensure high yield and purity.
Chemical Reactions Analysis
Types of Reactions: 3-Chlorothiobenzamide undergoes various chemical reactions, including:
Oxidation: This compound can be oxidized to form using oxidizing agents such as or .
Reduction: Reduction of this compound can yield using reducing agents like .
Substitution: The chlorine atom in this compound can be substituted with other nucleophiles such as or under appropriate conditions.
Common Reagents and Conditions:
Oxidation: Hydrogen peroxide, potassium permanganate.
Reduction: Lithium aluminum hydride, sodium borohydride.
Substitution: Amines, thiols, in the presence of a base or catalyst.
Major Products:
Oxidation: 3-Chlorobenzamide.
Reduction: 3-Chlorobenzylamine.
Substitution: Various substituted benzamides depending on the nucleophile used.
Scientific Research Applications
3-Chlorothiobenzamide has several applications in scientific research:
Chemistry: It is used as an intermediate in the synthesis of more complex organic compounds.
Biology: Studies have explored its potential as an inhibitor of certain enzymes.
Medicine: Research is ongoing to investigate its potential therapeutic properties.
Industry: It is used in the production of specialty chemicals and as a reagent in various chemical processes.
Comparison with Similar Compounds
2-Chlorothiobenzamide: Similar structure but with the chlorine atom at the second position.
3-Chlorobenzamide: Lacks the thiocarbonyl group.
3-Chlorobenzylamine: Resulting from the reduction of 3-Chlorothiobenzamide.
Uniqueness: this compound is unique due to the presence of both a chlorine atom and a thiocarbonyl group, which confer distinct chemical reactivity and biological activity compared to its analogs .
Properties
IUPAC Name |
3-chlorobenzenecarbothioamide | |
---|---|---|
Source | PubChem | |
URL | https://pubchem.ncbi.nlm.nih.gov | |
Description | Data deposited in or computed by PubChem | |
InChI |
InChI=1S/C7H6ClNS/c8-6-3-1-2-5(4-6)7(9)10/h1-4H,(H2,9,10) | |
Source | PubChem | |
URL | https://pubchem.ncbi.nlm.nih.gov | |
Description | Data deposited in or computed by PubChem | |
InChI Key |
OQEBJXXIPHYYEG-UHFFFAOYSA-N | |
Source | PubChem | |
URL | https://pubchem.ncbi.nlm.nih.gov | |
Description | Data deposited in or computed by PubChem | |
Canonical SMILES |
C1=CC(=CC(=C1)Cl)C(=S)N | |
Source | PubChem | |
URL | https://pubchem.ncbi.nlm.nih.gov | |
Description | Data deposited in or computed by PubChem | |
Molecular Formula |
C7H6ClNS | |
Source | PubChem | |
URL | https://pubchem.ncbi.nlm.nih.gov | |
Description | Data deposited in or computed by PubChem | |
DSSTOX Substance ID |
DTXSID30180162 | |
Record name | Benzenecarbothioamide, 3-chloro- | |
Source | EPA DSSTox | |
URL | https://comptox.epa.gov/dashboard/DTXSID30180162 | |
Description | DSSTox provides a high quality public chemistry resource for supporting improved predictive toxicology. | |
Molecular Weight |
171.65 g/mol | |
Source | PubChem | |
URL | https://pubchem.ncbi.nlm.nih.gov | |
Description | Data deposited in or computed by PubChem | |
CAS No. |
2548-79-0 | |
Record name | Benzenecarbothioamide, 3-chloro- | |
Source | ChemIDplus | |
URL | https://pubchem.ncbi.nlm.nih.gov/substance/?source=chemidplus&sourceid=0002548790 | |
Description | ChemIDplus is a free, web search system that provides access to the structure and nomenclature authority files used for the identification of chemical substances cited in National Library of Medicine (NLM) databases, including the TOXNET system. | |
Record name | Benzenecarbothioamide, 3-chloro- | |
Source | EPA DSSTox | |
URL | https://comptox.epa.gov/dashboard/DTXSID30180162 | |
Description | DSSTox provides a high quality public chemistry resource for supporting improved predictive toxicology. | |
Record name | 2548-79-0 | |
Source | European Chemicals Agency (ECHA) | |
URL | https://echa.europa.eu/information-on-chemicals | |
Description | The European Chemicals Agency (ECHA) is an agency of the European Union which is the driving force among regulatory authorities in implementing the EU's groundbreaking chemicals legislation for the benefit of human health and the environment as well as for innovation and competitiveness. | |
Explanation | Use of the information, documents and data from the ECHA website is subject to the terms and conditions of this Legal Notice, and subject to other binding limitations provided for under applicable law, the information, documents and data made available on the ECHA website may be reproduced, distributed and/or used, totally or in part, for non-commercial purposes provided that ECHA is acknowledged as the source: "Source: European Chemicals Agency, http://echa.europa.eu/". Such acknowledgement must be included in each copy of the material. ECHA permits and encourages organisations and individuals to create links to the ECHA website under the following cumulative conditions: Links can only be made to webpages that provide a link to the Legal Notice page. | |
Retrosynthesis Analysis
AI-Powered Synthesis Planning: Our tool employs the Template_relevance Pistachio, Template_relevance Bkms_metabolic, Template_relevance Pistachio_ringbreaker, Template_relevance Reaxys, Template_relevance Reaxys_biocatalysis model, leveraging a vast database of chemical reactions to predict feasible synthetic routes.
One-Step Synthesis Focus: Specifically designed for one-step synthesis, it provides concise and direct routes for your target compounds, streamlining the synthesis process.
Accurate Predictions: Utilizing the extensive PISTACHIO, BKMS_METABOLIC, PISTACHIO_RINGBREAKER, REAXYS, REAXYS_BIOCATALYSIS database, our tool offers high-accuracy predictions, reflecting the latest in chemical research and data.
Strategy Settings
Precursor scoring | Relevance Heuristic |
---|---|
Min. plausibility | 0.01 |
Model | Template_relevance |
Template Set | Pistachio/Bkms_metabolic/Pistachio_ringbreaker/Reaxys/Reaxys_biocatalysis |
Top-N result to add to graph | 6 |
Feasible Synthetic Routes
Disclaimer and Information on In-Vitro Research Products
Please be aware that all articles and product information presented on BenchChem are intended solely for informational purposes. The products available for purchase on BenchChem are specifically designed for in-vitro studies, which are conducted outside of living organisms. In-vitro studies, derived from the Latin term "in glass," involve experiments performed in controlled laboratory settings using cells or tissues. It is important to note that these products are not categorized as medicines or drugs, and they have not received approval from the FDA for the prevention, treatment, or cure of any medical condition, ailment, or disease. We must emphasize that any form of bodily introduction of these products into humans or animals is strictly prohibited by law. It is essential to adhere to these guidelines to ensure compliance with legal and ethical standards in research and experimentation.