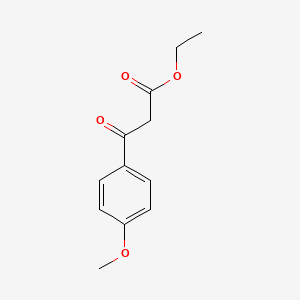
Ethyl 3-(4-methoxyphenyl)-3-oxopropanoate
Overview
Description
Ethyl 3-(4-methoxyphenyl)-3-oxopropanoate is an organic compound that belongs to the class of esters It is characterized by the presence of an ethyl ester group attached to a 3-oxopropanoate moiety, which is further substituted with a 4-methoxyphenyl group
Mechanism of Action
Target of Action
It’s worth noting that compounds with similar structures, such as indole derivatives, have been found to bind with high affinity to multiple receptors . These receptors play a crucial role in various biological activities, including antiviral, anti-inflammatory, anticancer, and antioxidant activities .
Mode of Action
For instance, indole derivatives, which share some structural similarities, have been shown to possess various biological activities .
Biochemical Pathways
Similar compounds, such as indole derivatives, have been found to affect a variety of biochemical pathways, leading to diverse biological activities .
Pharmacokinetics
A study on a structurally similar compound, 3-(4-hydroxy-3-methoxyphenyl)propionic acid, showed that it undergoes rapid metabolism and wide tissue distribution with a ≥12% absorption ratio .
Result of Action
Similar compounds have been shown to have various biological activities, including antiviral, anti-inflammatory, and anticancer activities .
Action Environment
For example, octyl methoxycinnamate, a compound used in sunscreens, is known to absorb UV-B rays from the sun, protecting the skin from damage .
Biochemical Analysis
Biochemical Properties
Ethyl 3-(4-methoxyphenyl)-3-oxopropanoate plays a significant role in biochemical reactions, particularly in the context of enzyme interactions. It is known to interact with enzymes such as esterases and lipases, which catalyze the hydrolysis of ester bonds. These interactions are crucial for the compound’s metabolic processing and its subsequent effects on cellular functions. Additionally, this compound can interact with proteins and other biomolecules, influencing their structure and function through non-covalent binding interactions .
Cellular Effects
This compound has been shown to affect various types of cells and cellular processes. It can influence cell signaling pathways, gene expression, and cellular metabolism. For instance, the compound has been observed to modulate the activity of key signaling molecules, leading to alterations in cellular responses. Furthermore, this compound can impact gene expression by interacting with transcription factors and other regulatory proteins, thereby affecting the transcriptional activity of specific genes .
Molecular Mechanism
The molecular mechanism of action of this compound involves its binding interactions with biomolecules. The compound can act as an enzyme inhibitor or activator, depending on the specific enzyme it interacts with. For example, it can inhibit the activity of certain esterases by binding to their active sites, preventing substrate access and subsequent catalysis. Additionally, this compound can induce changes in gene expression by modulating the activity of transcription factors and other regulatory proteins .
Temporal Effects in Laboratory Settings
In laboratory settings, the effects of this compound can change over time due to its stability and degradation. The compound is relatively stable under standard laboratory conditions, but it can undergo hydrolysis and other degradation processes over extended periods. These changes can influence its long-term effects on cellular function, as observed in both in vitro and in vivo studies. For instance, prolonged exposure to this compound can lead to alterations in cellular metabolism and gene expression .
Dosage Effects in Animal Models
The effects of this compound vary with different dosages in animal models. At low doses, the compound may exhibit minimal effects on cellular function, while higher doses can lead to significant biochemical and physiological changes. Threshold effects have been observed, where a certain dosage level is required to elicit a measurable response. Additionally, high doses of this compound can result in toxic or adverse effects, such as cellular damage and disruption of normal metabolic processes .
Metabolic Pathways
This compound is involved in various metabolic pathways, including those mediated by esterases and lipases. These enzymes catalyze the hydrolysis of the ester bond, resulting in the formation of 4-methoxyphenylacetic acid and ethanol. The compound can also interact with cofactors and other metabolic intermediates, influencing metabolic flux and metabolite levels. These interactions are essential for the compound’s metabolic processing and its subsequent effects on cellular function .
Transport and Distribution
Within cells and tissues, this compound is transported and distributed through various mechanisms. It can interact with transporters and binding proteins, facilitating its movement across cellular membranes and its localization within specific cellular compartments. The compound’s distribution can also be influenced by its physicochemical properties, such as solubility and lipophilicity, which affect its ability to traverse biological membranes .
Subcellular Localization
The subcellular localization of this compound is determined by its interactions with targeting signals and post-translational modifications. These interactions direct the compound to specific compartments or organelles within the cell, where it can exert its biochemical effects. For example, this compound may localize to the endoplasmic reticulum or mitochondria, influencing the function of these organelles and their associated metabolic processes .
Preparation Methods
Synthetic Routes and Reaction Conditions
The synthesis of Ethyl 3-(4-methoxyphenyl)-3-oxopropanoate can be achieved through several methods. One common approach involves the esterification of 3-(4-methoxyphenyl)-3-oxopropanoic acid with ethanol in the presence of a strong acid catalyst such as sulfuric acid. The reaction is typically carried out under reflux conditions to ensure complete conversion of the acid to the ester.
Another method involves the Claisen condensation reaction between ethyl acetate and 4-methoxybenzaldehyde in the presence of a strong base such as sodium ethoxide. This reaction forms the β-keto ester, which is then purified through recrystallization or distillation.
Industrial Production Methods
In an industrial setting, the production of this compound may involve continuous flow processes to enhance efficiency and yield. Catalysts such as sulfuric acid or p-toluenesulfonic acid are commonly used to facilitate the esterification reaction. The use of automated reactors and purification systems ensures consistent product quality and scalability.
Chemical Reactions Analysis
Types of Reactions
Ethyl 3-(4-methoxyphenyl)-3-oxopropanoate undergoes various chemical reactions, including:
Oxidation: The compound can be oxidized to form corresponding carboxylic acids or ketones.
Reduction: Reduction reactions can convert the ester group to an alcohol or the keto group to a hydroxyl group.
Substitution: Nucleophilic substitution reactions can occur at the ester or keto positions, leading to the formation of new derivatives.
Common Reagents and Conditions
Oxidation: Common oxidizing agents include potassium permanganate (KMnO₄) and chromium trioxide (CrO₃).
Reduction: Reducing agents such as lithium aluminum hydride (LiAlH₄) or sodium borohydride (NaBH₄) are used.
Substitution: Reagents like Grignard reagents (RMgX) or organolithium compounds (RLi) are employed for nucleophilic substitution.
Major Products
The major products formed from these reactions include:
- Carboxylic acids and ketones from oxidation.
- Alcohols from reduction.
- Various substituted derivatives from nucleophilic substitution.
Scientific Research Applications
Ethyl 3-(4-methoxyphenyl)-3-oxopropanoate has several scientific research applications:
Chemistry: It is used as an intermediate in the synthesis of more complex organic molecules.
Biology: The compound is studied for its potential biological activities, including antimicrobial and anti-inflammatory properties.
Medicine: Research is ongoing to explore its potential as a precursor for pharmaceutical compounds.
Industry: It is used in the production of fine chemicals and as a building block for various industrial products.
Comparison with Similar Compounds
Ethyl 3-(4-methoxyphenyl)-3-oxopropanoate can be compared with similar compounds such as:
Ethyl 2-cyano-3-(4-methoxyphenyl)acrylate: Both compounds contain a 4-methoxyphenyl group, but differ in their functional groups and reactivity.
Cyanoacetohydrazides: These compounds share similar structural features and are used in the synthesis of heterocyclic compounds.
Properties
IUPAC Name |
ethyl 3-(4-methoxyphenyl)-3-oxopropanoate | |
---|---|---|
Source | PubChem | |
URL | https://pubchem.ncbi.nlm.nih.gov | |
Description | Data deposited in or computed by PubChem | |
InChI |
InChI=1S/C12H14O4/c1-3-16-12(14)8-11(13)9-4-6-10(15-2)7-5-9/h4-7H,3,8H2,1-2H3 | |
Source | PubChem | |
URL | https://pubchem.ncbi.nlm.nih.gov | |
Description | Data deposited in or computed by PubChem | |
InChI Key |
KRAHENMBSVAAHD-UHFFFAOYSA-N | |
Source | PubChem | |
URL | https://pubchem.ncbi.nlm.nih.gov | |
Description | Data deposited in or computed by PubChem | |
Canonical SMILES |
CCOC(=O)CC(=O)C1=CC=C(C=C1)OC | |
Source | PubChem | |
URL | https://pubchem.ncbi.nlm.nih.gov | |
Description | Data deposited in or computed by PubChem | |
Molecular Formula |
C12H14O4 | |
Source | PubChem | |
URL | https://pubchem.ncbi.nlm.nih.gov | |
Description | Data deposited in or computed by PubChem | |
DSSTOX Substance ID |
DTXSID5062683 | |
Record name | Benzenepropanoic acid, 4-methoxy-.beta.-oxo-, ethyl ester | |
Source | EPA DSSTox | |
URL | https://comptox.epa.gov/dashboard/DTXSID5062683 | |
Description | DSSTox provides a high quality public chemistry resource for supporting improved predictive toxicology. | |
Molecular Weight |
222.24 g/mol | |
Source | PubChem | |
URL | https://pubchem.ncbi.nlm.nih.gov | |
Description | Data deposited in or computed by PubChem | |
CAS No. |
2881-83-6 | |
Record name | Ethyl 3-(4-methoxyphenyl)-3-oxopropanoate | |
Source | CAS Common Chemistry | |
URL | https://commonchemistry.cas.org/detail?cas_rn=2881-83-6 | |
Description | CAS Common Chemistry is an open community resource for accessing chemical information. Nearly 500,000 chemical substances from CAS REGISTRY cover areas of community interest, including common and frequently regulated chemicals, and those relevant to high school and undergraduate chemistry classes. This chemical information, curated by our expert scientists, is provided in alignment with our mission as a division of the American Chemical Society. | |
Explanation | The data from CAS Common Chemistry is provided under a CC-BY-NC 4.0 license, unless otherwise stated. | |
Record name | Ethyl p-anisoylacetate | |
Source | ChemIDplus | |
URL | https://pubchem.ncbi.nlm.nih.gov/substance/?source=chemidplus&sourceid=0002881836 | |
Description | ChemIDplus is a free, web search system that provides access to the structure and nomenclature authority files used for the identification of chemical substances cited in National Library of Medicine (NLM) databases, including the TOXNET system. | |
Record name | Ethyl p-anisoylacetate | |
Source | DTP/NCI | |
URL | https://dtp.cancer.gov/dtpstandard/servlet/dwindex?searchtype=NSC&outputformat=html&searchlist=69701 | |
Description | The NCI Development Therapeutics Program (DTP) provides services and resources to the academic and private-sector research communities worldwide to facilitate the discovery and development of new cancer therapeutic agents. | |
Explanation | Unless otherwise indicated, all text within NCI products is free of copyright and may be reused without our permission. Credit the National Cancer Institute as the source. | |
Record name | Benzenepropanoic acid, 4-methoxy-.beta.-oxo-, ethyl ester | |
Source | EPA Chemicals under the TSCA | |
URL | https://www.epa.gov/chemicals-under-tsca | |
Description | EPA Chemicals under the Toxic Substances Control Act (TSCA) collection contains information on chemicals and their regulations under TSCA, including non-confidential content from the TSCA Chemical Substance Inventory and Chemical Data Reporting. | |
Record name | Benzenepropanoic acid, 4-methoxy-.beta.-oxo-, ethyl ester | |
Source | EPA DSSTox | |
URL | https://comptox.epa.gov/dashboard/DTXSID5062683 | |
Description | DSSTox provides a high quality public chemistry resource for supporting improved predictive toxicology. | |
Record name | Ethyl p-anisoylacetate | |
Source | European Chemicals Agency (ECHA) | |
URL | https://echa.europa.eu/substance-information/-/substanceinfo/100.018.849 | |
Description | The European Chemicals Agency (ECHA) is an agency of the European Union which is the driving force among regulatory authorities in implementing the EU's groundbreaking chemicals legislation for the benefit of human health and the environment as well as for innovation and competitiveness. | |
Explanation | Use of the information, documents and data from the ECHA website is subject to the terms and conditions of this Legal Notice, and subject to other binding limitations provided for under applicable law, the information, documents and data made available on the ECHA website may be reproduced, distributed and/or used, totally or in part, for non-commercial purposes provided that ECHA is acknowledged as the source: "Source: European Chemicals Agency, http://echa.europa.eu/". Such acknowledgement must be included in each copy of the material. ECHA permits and encourages organisations and individuals to create links to the ECHA website under the following cumulative conditions: Links can only be made to webpages that provide a link to the Legal Notice page. | |
Record name | ETHYL P-ANISOYLACETATE | |
Source | FDA Global Substance Registration System (GSRS) | |
URL | https://gsrs.ncats.nih.gov/ginas/app/beta/substances/97LJ3S7ZBW | |
Description | The FDA Global Substance Registration System (GSRS) enables the efficient and accurate exchange of information on what substances are in regulated products. Instead of relying on names, which vary across regulatory domains, countries, and regions, the GSRS knowledge base makes it possible for substances to be defined by standardized, scientific descriptions. | |
Explanation | Unless otherwise noted, the contents of the FDA website (www.fda.gov), both text and graphics, are not copyrighted. They are in the public domain and may be republished, reprinted and otherwise used freely by anyone without the need to obtain permission from FDA. Credit to the U.S. Food and Drug Administration as the source is appreciated but not required. | |
Synthesis routes and methods I
Procedure details
Synthesis routes and methods II
Procedure details
Synthesis routes and methods III
Procedure details
Retrosynthesis Analysis
AI-Powered Synthesis Planning: Our tool employs the Template_relevance Pistachio, Template_relevance Bkms_metabolic, Template_relevance Pistachio_ringbreaker, Template_relevance Reaxys, Template_relevance Reaxys_biocatalysis model, leveraging a vast database of chemical reactions to predict feasible synthetic routes.
One-Step Synthesis Focus: Specifically designed for one-step synthesis, it provides concise and direct routes for your target compounds, streamlining the synthesis process.
Accurate Predictions: Utilizing the extensive PISTACHIO, BKMS_METABOLIC, PISTACHIO_RINGBREAKER, REAXYS, REAXYS_BIOCATALYSIS database, our tool offers high-accuracy predictions, reflecting the latest in chemical research and data.
Strategy Settings
Precursor scoring | Relevance Heuristic |
---|---|
Min. plausibility | 0.01 |
Model | Template_relevance |
Template Set | Pistachio/Bkms_metabolic/Pistachio_ringbreaker/Reaxys/Reaxys_biocatalysis |
Top-N result to add to graph | 6 |
Feasible Synthetic Routes
Disclaimer and Information on In-Vitro Research Products
Please be aware that all articles and product information presented on BenchChem are intended solely for informational purposes. The products available for purchase on BenchChem are specifically designed for in-vitro studies, which are conducted outside of living organisms. In-vitro studies, derived from the Latin term "in glass," involve experiments performed in controlled laboratory settings using cells or tissues. It is important to note that these products are not categorized as medicines or drugs, and they have not received approval from the FDA for the prevention, treatment, or cure of any medical condition, ailment, or disease. We must emphasize that any form of bodily introduction of these products into humans or animals is strictly prohibited by law. It is essential to adhere to these guidelines to ensure compliance with legal and ethical standards in research and experimentation.