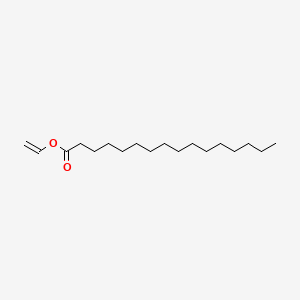
Vinyl palmitate
Overview
Description
Vinyl palmitate (C₁₆H₃₀O₂) is a long-chain vinyl ester derived from palmitic acid (C16:0) and vinyl alcohol. It is widely utilized in organic synthesis, particularly in esterification and transesterification reactions, to introduce hydrophobic palmitoyl groups into substrates such as cellulose, peptides, and sugars . Its nonpolar nature and steric bulk make it advantageous for modifying material properties like hydrophobicity and thermal stability. Applications span polymer chemistry (e.g., cellulose derivatives), pharmaceutical lipidation (e.g., peptide therapeutics), and enzymatic synthesis of glycolipids .
Preparation Methods
Synthetic Routes and Reaction Conditions: Vinyl palmitate can be synthesized through the esterification of palmitic acid with vinyl alcohol. This reaction typically requires a catalyst, such as sulfuric acid, to proceed efficiently. The reaction conditions often involve heating the reactants under reflux to achieve a high yield of the ester.
Industrial Production Methods: In industrial settings, this compound is commonly produced via transesterification. One method involves the reaction of palmitic acid with vinyl acetate in the presence of a catalyst like mercuric acetate and sulfuric acid. The reaction is carried out under reflux conditions, and the excess vinyl acetate is removed by distillation .
Chemical Reactions Analysis
Types of Reactions: Vinyl palmitate undergoes various chemical reactions, including:
Transesterification: This reaction involves the exchange of the ester group with another alcohol.
Hydrolysis: In the presence of water and an acid or base catalyst, this compound can hydrolyze to produce palmitic acid and vinyl alcohol.
Polymerization: this compound can undergo polymerization reactions to form polyvinyl esters, which are useful in the production of various polymers.
Common Reagents and Conditions:
Catalysts: Sulfuric acid, mercuric acetate, and lipases (enzymes) are commonly used as catalysts in the reactions involving this compound
Solvents: Dimethyl sulfoxide (DMSO) and ionic liquids are often used as solvents to facilitate the reactions
Major Products Formed:
Monopalmitin: Formed through transesterification with glycerol.
Palmitic Acid: Produced via hydrolysis of this compound.
Scientific Research Applications
Vinyl palmitate has several applications in scientific research:
Mechanism of Action
The mechanism of action of vinyl palmitate primarily involves its reactivity as an ester. In enzymatic reactions, lipases catalyze the transesterification of this compound with other alcohols, leading to the formation of monoacylglycerols. The molecular targets in these reactions are the ester bonds, which are cleaved and reformed during the process .
Comparison with Similar Compounds
Chain Length and Reactivity
The alkyl chain length of vinyl esters significantly impacts reactivity. Shorter-chain esters (e.g., vinyl butyrate, C4) exhibit higher reaction rates due to lower steric hindrance and higher polarity. For example:
- O–C=O Bond Concentration: In nanocellulose film esterification, vinyl butyrate (C4) showed an O–C=O bond concentration of 4.2%, while vinyl palmitate (C16) had only 2.5%, indicating slower reaction kinetics with longer chains .
- Conversion Rates : In transesterification reactions for puerarin palmitate synthesis, this compound achieved a 30% conversion rate, underperforming compared to palmitic anhydride (55.5%) due to steric limitations .
Table 1: O–C=O Bond Concentration by Alkyl Chain Length
Vinyl Ester | Chain Length | O–C=O Bond Concentration (%) |
---|---|---|
Vinyl butyrate | C4 | 4.2 |
Vinyl octanoate | C8 | 3.4 (with UV/O3 pretreatment) |
Vinyl laurate | C12 | 2.8–3.4 |
This compound | C16 | 2.5 |
Substitution Efficiency in Cellulose Derivatives
This compound outperforms shorter-chain esters in acetylation efficiency when modifying cellulose in ionic liquids. The degree of substitution (DS) correlates with chain length and steric effects:
- DSacetate: this compound achieved a DSacetate of 0.4 (20.0% contribution), surpassing vinyl laurate (C12, 11.1%) and octanoate (C8, 6.3%) .
- Steric Demand: Branched esters like vinyl 2-ethylhexyloate (C8, branched) showed low DSacyl donor (0.9) due to steric hindrance, highlighting this compound’s balance of nonpolarity and manageable bulk .
Table 2: Substitution Degrees of Cellulose Esters
Acyl Donor | DSacyl donor | DSacetate | %-DSacetate |
---|---|---|---|
Vinyl octanoate (C8) | 1.5 | 0.1 | 6.3 |
Vinyl laurate (C12) | 1.6 | 0.2 | 11.1 |
This compound (C16) | 1.6 | 0.4 | 20.0 |
Vinyl 2-ethylhexyloate | 0.9 | 0.2 | 18.1 |
Solvent and Reaction Conditions
Reaction efficiency depends on solvent polarity and water content:
- Glucose Palmitate Synthesis : In acetone, this compound achieved 90% conversion to 6-O-glucose palmitate with molecular sieves, whereas dioxane required water removal for similar yields .
- UV/O3 Pretreatment : Enhanced reactivity for vinyl laurate (C12) but reduced efficiency for this compound, likely due to oxidative degradation of long chains .
Conversion Efficiency in Transesterification
This compound’s performance varies by reaction type:
- Puerarin Palmitate : 30% conversion rate, lower than palmitic anhydride (55.5%) due to slower kinetics .
- Sucrose Esters: Base-catalyzed transesterification with this compound yielded >85% monoesters, comparable to commercial derivatives .
Table 3: Conversion Rates of Acyl Donors
Acyl Donor | Conversion Rate (%) |
---|---|
Palmitic Anhydride | 55.5 |
This compound | 30.0 |
Biological Activity
Vinyl palmitate, a fatty acid ester derived from palmitic acid, has garnered attention for its biological activities and potential applications in various fields, including food science, pharmaceuticals, and biochemistry. This article explores the biological activity of this compound, highlighting its synthesis, antimicrobial properties, enzymatic reactions, and potential therapeutic applications.
This compound is synthesized through the esterification of palmitic acid with vinyl alcohol or via lipase-catalyzed reactions. The compound exhibits amphiphilic properties, making it suitable for various applications, including as a surfactant and emulsifier in food products.
Table 1: Synthesis Methods of this compound
Method | Description | Reference |
---|---|---|
Lipase-Catalyzed Esterification | Utilizes lipases to catalyze the reaction in organic solvents | |
Direct Esterification | Involves reacting palmitic acid with vinyl alcohol |
2. Microbial Inhibitory Properties
Research indicates that this compound possesses antimicrobial properties against food-related microorganisms. Studies have shown that it can inhibit the growth of various bacteria, making it a potential candidate for food preservation.
Case Study: Antimicrobial Activity
In a comparative study of maltodextrin fatty acid esters, this compound exhibited significant microbial inhibitory effects against pathogens such as Listeria monocytogenes and Escherichia coli. The effectiveness was attributed to its ability to disrupt microbial cell membranes.
Table 2: Antimicrobial Efficacy of this compound
Microorganism | Minimum Inhibitory Concentration (MIC) | Reference |
---|---|---|
Listeria monocytogenes | 0.5% | |
Escherichia coli | 0.3% | |
Salmonella spp. | 0.4% |
3. Enzymatic Reactions and Kinetics
The enzymatic synthesis of this compound has been extensively studied, particularly regarding its reaction kinetics in various solvents. The use of lipases from different sources has shown varying efficiencies in catalyzing the formation of this compound.
Table 3: Kinetic Parameters of Lipase-Catalyzed Reactions
Lipase Source | Optimal Solvent | Conversion Rate (μmol.min⁻¹.g⁻¹) | Reference |
---|---|---|---|
Candida antarctica B (CALB) | Acetone | 93 | |
Thermomyces lanuginosus (TLL) | Acetonitrile | 74 | |
Rhizopus oryzae (RML) | Tert-butanol | 60 |
4. Therapeutic Applications
This compound's biological activity extends to potential therapeutic applications. Studies have indicated its cytotoxic effects on certain cancer cell lines, suggesting a role in cancer treatment.
Case Study: Antitumor Activity
Research demonstrated that this compound exhibited selective cytotoxicity against human leukemic cells at specific concentrations (12.5 to 50 μg/ml), indicating its potential as an anticancer agent.
5. Conclusion
This compound is a versatile compound with significant biological activity, particularly in antimicrobial properties and potential therapeutic applications. Its synthesis through enzymatic processes offers a sustainable approach to producing this compound for various applications in food science and medicine.
Q & A
Basic Research Questions
Q. What are the standard protocols for synthesizing vinyl palmitate in laboratory settings, and how can purity be validated?
To synthesize this compound, esterification of palmitic acid with vinyl alcohol (or transesterification with vinyl acetate) is common. Reaction conditions (e.g., acid catalysis, temperature control at 60–80°C, inert atmosphere) must be optimized to minimize side reactions like hydrolysis . Purity validation requires gas chromatography (GC) or high-performance liquid chromatography (HPLC) coupled with mass spectrometry (MS) to confirm molecular weight and detect impurities. Nuclear magnetic resonance (NMR) spectroscopy (¹H and ¹³C) is critical for structural confirmation, particularly to verify ester bond formation and vinyl group integrity .
Q. How should researchers design experiments to characterize the physicochemical properties of this compound?
Key properties include melting point, solubility, and thermal stability. Differential scanning calorimetry (DSC) determines melting behavior, while thermogravimetric analysis (TGA) assesses thermal decomposition. Solubility studies should use a range of solvents (polar, nonpolar) under controlled temperatures. Surface tension and viscosity measurements may require rheometers or tensiometers. Ensure replicates (n ≥ 3) and statistical analysis (e.g., ANOVA) to account for variability .
Q. What safety protocols are essential when handling this compound in laboratory environments?
Use nitrile gloves and safety goggles to prevent skin/eye contact. Work in a fume hood to avoid inhalation of vapors. Store in airtight containers away from oxidizers. In case of spills, neutralize with inert absorbents (e.g., vermiculite) and dispose of according to hazardous waste regulations. Safety data sheets (SDS) must be reviewed for emergency procedures .
Advanced Research Questions
Q. How can factorial design be applied to optimize reaction conditions for this compound synthesis?
A 2<sup>k</sup> factorial design evaluates variables like catalyst concentration, temperature, and reaction time. For example, two levels (high/low) of each factor are tested to identify interactions affecting yield. Response surface methodology (RSM) further refines optimal conditions. Statistical software (e.g., Minitab, R) analyzes main effects and interactions, ensuring robust process scalability .
Q. What methodologies resolve contradictions in reported data on this compound’s stability under oxidative conditions?
Conflicting stability data may arise from differences in experimental setups (e.g., oxygen exposure, light sources). To resolve this, design controlled oxidative stress tests using accelerated aging protocols with UV irradiation or peroxide exposure. Monitor degradation via Fourier-transform infrared spectroscopy (FTIR) for carbonyl index changes and GC-MS to identify breakdown products. Replicate studies across independent labs to validate findings .
Q. How can researchers investigate the role of this compound in lipid-based drug delivery systems using in vitro models?
Develop lipid nanoparticles (e.g., solid lipid nanoparticles, SLNs) incorporating this compound. Characterize particle size (dynamic light scattering), zeta potential (electrophoretic mobility), and encapsulation efficiency (ultracentrifugation followed by HPLC). Use cell culture models (e.g., Caco-2 for intestinal absorption) to assess bioavailability. Compare release kinetics in simulated physiological fluids (e.g., PBS at pH 6.8) using dialysis membranes .
Q. What theoretical frameworks guide mechanistic studies of this compound’s interactions with biological membranes?
Molecular dynamics (MD) simulations based on coarse-grained or all-atom models predict interactions between this compound and lipid bilayers. Experimental validation via fluorescence anisotropy (to measure membrane fluidity) and Langmuir trough experiments (to assess monolayer penetration) can corroborate computational findings. Link results to existing theories like the “flip-flop” mechanism or partition coefficients .
Q. Methodological Considerations
Q. How should researchers address reproducibility challenges in this compound studies?
Document all parameters rigorously: reagent sources, equipment calibration, and environmental conditions (humidity, temperature). Use internal standards in analytical methods (e.g., deuterated analogs in GC-MS). Share raw data and protocols via repositories like Zenodo or Figshare to enable cross-validation .
Q. What statistical approaches are recommended for analyzing dose-response relationships in this compound toxicity assays?
Nonlinear regression models (e.g., log-logistic curves) estimate EC50 values. Use software like GraphPad Prism for curve fitting and confidence interval calculations. For high-throughput data, apply machine learning algorithms (e.g., random forests) to identify toxicity predictors .
Q. How can mixed-methods research frameworks integrate qualitative and quantitative data in this compound applications?
Combine quantitative metrics (e.g., bioavailability) with qualitative stakeholder interviews (e.g., pharmacologists’ perceptions of formulation feasibility). Use triangulation to reconcile discrepancies. Code interview transcripts with NVivo and correlate themes with experimental outcomes .
Q. Data Integrity and Reporting Standards
- Material Characterization : Report NMR shifts (δ ppm), GC retention times, and MS fragmentation patterns in supplementary files .
- Ethical Compliance : Disclose funding sources and conflicts of interest. For human cell studies, include ethics committee approval codes .
- Replication : Follow CONSORT or ARRIVE guidelines for experimental design transparency .
Properties
IUPAC Name |
ethenyl hexadecanoate | |
---|---|---|
Source | PubChem | |
URL | https://pubchem.ncbi.nlm.nih.gov | |
Description | Data deposited in or computed by PubChem | |
InChI |
InChI=1S/C18H34O2/c1-3-5-6-7-8-9-10-11-12-13-14-15-16-17-18(19)20-4-2/h4H,2-3,5-17H2,1H3 | |
Source | PubChem | |
URL | https://pubchem.ncbi.nlm.nih.gov | |
Description | Data deposited in or computed by PubChem | |
InChI Key |
UJRIYYLGNDXVTA-UHFFFAOYSA-N | |
Source | PubChem | |
URL | https://pubchem.ncbi.nlm.nih.gov | |
Description | Data deposited in or computed by PubChem | |
Canonical SMILES |
CCCCCCCCCCCCCCCC(=O)OC=C | |
Source | PubChem | |
URL | https://pubchem.ncbi.nlm.nih.gov | |
Description | Data deposited in or computed by PubChem | |
Molecular Formula |
C18H34O2 | |
Source | PubChem | |
URL | https://pubchem.ncbi.nlm.nih.gov | |
Description | Data deposited in or computed by PubChem | |
Related CAS |
30398-71-1 | |
Record name | Hexadecanoic acid, ethenyl ester, homopolymer | |
Source | CAS Common Chemistry | |
URL | https://commonchemistry.cas.org/detail?cas_rn=30398-71-1 | |
Description | CAS Common Chemistry is an open community resource for accessing chemical information. Nearly 500,000 chemical substances from CAS REGISTRY cover areas of community interest, including common and frequently regulated chemicals, and those relevant to high school and undergraduate chemistry classes. This chemical information, curated by our expert scientists, is provided in alignment with our mission as a division of the American Chemical Society. | |
Explanation | The data from CAS Common Chemistry is provided under a CC-BY-NC 4.0 license, unless otherwise stated. | |
DSSTOX Substance ID |
DTXSID3061005 | |
Record name | Hexadecanoic acid, ethenyl ester | |
Source | EPA DSSTox | |
URL | https://comptox.epa.gov/dashboard/DTXSID3061005 | |
Description | DSSTox provides a high quality public chemistry resource for supporting improved predictive toxicology. | |
Molecular Weight |
282.5 g/mol | |
Source | PubChem | |
URL | https://pubchem.ncbi.nlm.nih.gov | |
Description | Data deposited in or computed by PubChem | |
CAS No. |
693-38-9 | |
Record name | Vinyl palmitate | |
Source | CAS Common Chemistry | |
URL | https://commonchemistry.cas.org/detail?cas_rn=693-38-9 | |
Description | CAS Common Chemistry is an open community resource for accessing chemical information. Nearly 500,000 chemical substances from CAS REGISTRY cover areas of community interest, including common and frequently regulated chemicals, and those relevant to high school and undergraduate chemistry classes. This chemical information, curated by our expert scientists, is provided in alignment with our mission as a division of the American Chemical Society. | |
Explanation | The data from CAS Common Chemistry is provided under a CC-BY-NC 4.0 license, unless otherwise stated. | |
Record name | Hexadecanoic acid, ethenyl ester | |
Source | ChemIDplus | |
URL | https://pubchem.ncbi.nlm.nih.gov/substance/?source=chemidplus&sourceid=0000693389 | |
Description | ChemIDplus is a free, web search system that provides access to the structure and nomenclature authority files used for the identification of chemical substances cited in National Library of Medicine (NLM) databases, including the TOXNET system. | |
Record name | Hexadecanoic acid, ethenyl ester | |
Source | EPA Chemicals under the TSCA | |
URL | https://www.epa.gov/chemicals-under-tsca | |
Description | EPA Chemicals under the Toxic Substances Control Act (TSCA) collection contains information on chemicals and their regulations under TSCA, including non-confidential content from the TSCA Chemical Substance Inventory and Chemical Data Reporting. | |
Record name | Hexadecanoic acid, ethenyl ester | |
Source | EPA DSSTox | |
URL | https://comptox.epa.gov/dashboard/DTXSID3061005 | |
Description | DSSTox provides a high quality public chemistry resource for supporting improved predictive toxicology. | |
Record name | Vinyl palmitate | |
Source | European Chemicals Agency (ECHA) | |
URL | https://echa.europa.eu/substance-information/-/substanceinfo/100.010.684 | |
Description | The European Chemicals Agency (ECHA) is an agency of the European Union which is the driving force among regulatory authorities in implementing the EU's groundbreaking chemicals legislation for the benefit of human health and the environment as well as for innovation and competitiveness. | |
Explanation | Use of the information, documents and data from the ECHA website is subject to the terms and conditions of this Legal Notice, and subject to other binding limitations provided for under applicable law, the information, documents and data made available on the ECHA website may be reproduced, distributed and/or used, totally or in part, for non-commercial purposes provided that ECHA is acknowledged as the source: "Source: European Chemicals Agency, http://echa.europa.eu/". Such acknowledgement must be included in each copy of the material. ECHA permits and encourages organisations and individuals to create links to the ECHA website under the following cumulative conditions: Links can only be made to webpages that provide a link to the Legal Notice page. | |
Record name | Vinyl palmitate | |
Source | FDA Global Substance Registration System (GSRS) | |
URL | https://gsrs.ncats.nih.gov/ginas/app/beta/substances/J44FD9L9CY | |
Description | The FDA Global Substance Registration System (GSRS) enables the efficient and accurate exchange of information on what substances are in regulated products. Instead of relying on names, which vary across regulatory domains, countries, and regions, the GSRS knowledge base makes it possible for substances to be defined by standardized, scientific descriptions. | |
Explanation | Unless otherwise noted, the contents of the FDA website (www.fda.gov), both text and graphics, are not copyrighted. They are in the public domain and may be republished, reprinted and otherwise used freely by anyone without the need to obtain permission from FDA. Credit to the U.S. Food and Drug Administration as the source is appreciated but not required. | |
Retrosynthesis Analysis
AI-Powered Synthesis Planning: Our tool employs the Template_relevance Pistachio, Template_relevance Bkms_metabolic, Template_relevance Pistachio_ringbreaker, Template_relevance Reaxys, Template_relevance Reaxys_biocatalysis model, leveraging a vast database of chemical reactions to predict feasible synthetic routes.
One-Step Synthesis Focus: Specifically designed for one-step synthesis, it provides concise and direct routes for your target compounds, streamlining the synthesis process.
Accurate Predictions: Utilizing the extensive PISTACHIO, BKMS_METABOLIC, PISTACHIO_RINGBREAKER, REAXYS, REAXYS_BIOCATALYSIS database, our tool offers high-accuracy predictions, reflecting the latest in chemical research and data.
Strategy Settings
Precursor scoring | Relevance Heuristic |
---|---|
Min. plausibility | 0.01 |
Model | Template_relevance |
Template Set | Pistachio/Bkms_metabolic/Pistachio_ringbreaker/Reaxys/Reaxys_biocatalysis |
Top-N result to add to graph | 6 |
Feasible Synthetic Routes
Disclaimer and Information on In-Vitro Research Products
Please be aware that all articles and product information presented on BenchChem are intended solely for informational purposes. The products available for purchase on BenchChem are specifically designed for in-vitro studies, which are conducted outside of living organisms. In-vitro studies, derived from the Latin term "in glass," involve experiments performed in controlled laboratory settings using cells or tissues. It is important to note that these products are not categorized as medicines or drugs, and they have not received approval from the FDA for the prevention, treatment, or cure of any medical condition, ailment, or disease. We must emphasize that any form of bodily introduction of these products into humans or animals is strictly prohibited by law. It is essential to adhere to these guidelines to ensure compliance with legal and ethical standards in research and experimentation.