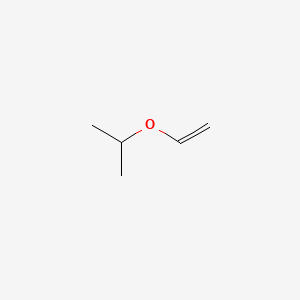
Vinyl isopropyl ether
Overview
Description
Vinyl isopropyl ether (VIPE; CAS 926-65-8) is a branched aliphatic vinyl ether with the chemical formula C₅H₁₀O. Structurally, it consists of an isopropyl group (-O-CH(CH₃)₂) attached to a vinyl moiety (CH₂=CH-). VIPE is synthesized via the Reppe process, involving the reaction of acetylene with isopropyl alcohol under high pressure and catalytic conditions . It is widely utilized in polymer chemistry as a reactive monomer, in coatings for its low glass transition temperature (Tg), and in radiation-curable adhesives due to its rapid polymerization kinetics .
Preparation Methods
Preparation Methods Overview
Vinyl isopropyl ether can be synthesized primarily by the reaction of isopropanol (2-propanol) with acetylene or via other vinyl ether synthesis routes. The key preparation methods include:
- Acid-catalyzed elimination of alcohols
- Reaction of acetylene with isopropanol
- Williamson ether synthesis (less common for vinyl ethers)
- Advanced catalytic processes involving acetal intermediates
Acid-Catalyzed Elimination of Alcohols
This classical method involves the dehydration of isopropanol in the presence of a strong acid catalyst (e.g., sulfuric acid). The acid catalyzes the elimination of water from the alcohol, forming the vinyl ether double bond:
$$
\text{(CH}3)2CHOH \xrightarrow[\text{acid}]{\text{heat}} \text{CH}2=CH-O-(CH(CH3)_2)
$$
- Simple reagents and setup
- Applicable to simple vinyl ethers
- Side reactions such as polymerization or degradation of vinyl ether
- Difficult to control purity and yield for this compound specifically
This method is less favored industrially for this compound due to these challenges.
Reaction of Acetylene with Isopropanol
The most widely used and industrially relevant method for this compound synthesis is the direct vinylation of isopropanol with acetylene gas in the presence of a base catalyst, typically potassium hydroxide (KOH).
$$
\text{C}2\text{H}2 + (CH3)2CHOH \xrightarrow[\text{KOH}]{\text{heat, pressure}} \text{CH}2=CH-O-(CH(CH3)_2)
$$
- Conducted at elevated temperatures (160–200 °C) and near atmospheric pressure
- Uses commercial-grade catalysts like hydrous KOH without prior purification
- Reaction medium can be a higher alcohol or its vinyl ether to facilitate catalyst dispersion and product separation
- Continuous or batch operation possible
- Vinyl ether product vaporizes and is separated by fractional distillation from unreacted acetylene and alcohol
- Continuous flow reactors maintain optimal temperature and reactant ratios to maximize yield and purity
- Catalyst attrition is low, and catalyst recycling is feasible
- Reaction medium and catalyst system allow switching between different vinyl ethers without extensive equipment changes
- Conversion rates of acetylene to vinyl ether can reach approximately 60–80% under optimized conditions
- Catalyst concentration typically 5–15% by weight relative to the reaction medium
- Reaction medium choice affects catalyst solubility and suspension, influencing reaction rates and selectivity
This method is the backbone of commercial this compound production due to its efficiency and scalability.
Williamson Ether Synthesis (Limited Use)
The Williamson ether synthesis involves reacting an alkoxide ion with a vinyl halide to form vinyl ethers:
$$
\text{(CH}3)2CHO^- + \text{CH}2=CH-X \rightarrow \text{CH}2=CH-O-(CH(CH3)2) + X^-
$$
While versatile, this method is less commonly applied for this compound due to:
- The difficulty in obtaining suitable vinyl halides
- Side reactions and lower atom economy compared to acetylene vinylation
It is more commonly used for other ether types.
Advanced Catalytic Processes Involving Acetal Intermediates
Recent patented processes have introduced methods to improve the purity and recovery of vinyl ethers, including this compound, by converting unreacted alcohol into acetals, which facilitates separation and recycling.
- After vinyl ether synthesis, residual isopropanol is converted into an acetal by adding a small amount of acid catalyst
- This conversion suppresses polymerization of vinyl ether during distillation
- The acetal and vinyl ether form separate fractions, enabling easier purification of high-purity vinyl ether
- Acetal can be decomposed back into alcohol and vinyl ether for recycling, improving overall process efficiency
Step No. | Description | Conditions/Notes |
---|---|---|
1 | Vinyl ether synthesis | Reaction of isopropanol with acetylene |
2 | Catalyst removal | Removal of base catalyst residues |
3 | Acetal formation | Acid-catalyzed conversion of residual alcohol |
4 | Distillation and purification | Separation of vinyl ether from acetal and impurities |
5 | Acetal decomposition and recycling | Recovery of alcohol and vinyl ether |
This method is especially useful when vinyl ether and alcohol form azeotropic mixtures, complicating direct distillation.
Comparative Data Table of Preparation Methods
Preparation Method | Catalyst/Conditions | Advantages | Limitations | Industrial Use |
---|---|---|---|---|
Acid-Catalyzed Elimination | Strong acid (H2SO4), heat | Simple reagents | Side reactions, low selectivity | Limited |
Acetylene + Isopropanol Vinylation | KOH catalyst, 160–200 °C, atmospheric pressure | High yield, scalable, continuous operation | Requires acetylene handling | Widely used |
Williamson Ether Synthesis | Alkoxide + vinyl halide, base | Selective for some ethers | Vinyl halide availability issues | Limited |
Acetal Intermediate Process | Acid catalyst for acetal formation | High purity, efficient recovery | Additional process steps | Emerging industrial use |
Research Findings and Optimization
- The vinylation of isopropanol with acetylene is optimized by controlling temperature, pressure, and catalyst concentration to balance conversion and selectivity.
- Using a reaction medium of higher alcohol or its vinyl ether improves catalyst dispersion and product separation.
- The acetal intermediate method enhances purification, allowing recovery of vinyl ether with minimal polymerization and by-products.
- Continuous flow reactors and vapor-phase reactant feeding improve reaction control and scalability.
Chemical Reactions Analysis
Types of Reactions
Vinyl isopropyl ether undergoes various chemical reactions, including:
Oxidation: The compound can be oxidized to form corresponding aldehydes or ketones.
Reduction: Reduction reactions can convert the vinyl ether to the corresponding alcohol.
Substitution: The vinyl group can undergo substitution reactions with various nucleophiles.
Common Reagents and Conditions
Oxidation: Common oxidizing agents include potassium permanganate (KMnO4) and chromium trioxide (CrO3).
Reduction: Reducing agents such as lithium aluminum hydride (LiAlH4) and sodium borohydride (NaBH4) are commonly used.
Substitution: Nucleophiles like halides, amines, and thiols can react with the vinyl group under mild conditions.
Major Products Formed
Oxidation: Aldehydes or ketones.
Reduction: Alcohols.
Substitution: Various substituted ethers depending on the nucleophile used.
Scientific Research Applications
Polymerization Reactions
Vinyl isopropyl ether is primarily utilized in cationic polymerization processes. It serves as a monomer for creating various polymers with desirable mechanical and thermal properties.
- Cationic Polymerization : VIP undergoes cationic polymerization readily, allowing for the synthesis of isotactic poly(vinyl ether)s. This process can be initiated using electrophilic catalysts, leading to polymers with high degrees of isotacticity and enhanced physical properties .
- Co-polymerization : VIP can be copolymerized with other vinyl monomers, resulting in materials with tailored properties for specific applications, such as adhesives and coatings .
Synthesis of Biologically Active Molecules
In the field of medicinal chemistry, this compound is employed in the synthesis of biologically active compounds and pharmaceuticals. Its reactivity allows for the formation of complex structures that are crucial in drug development .
Industrial Applications
VIP is used as a low-viscosity monomer in various industrial applications:
- Adhesives and Coatings : this compound contributes to the formulation of adhesives and coatings that require rapid curing and strong adhesion properties. It is particularly effective in weather-resistant coatings for construction materials .
- Lubricants and Greases : The compound's properties make it suitable for use in lubricants, enhancing their performance under varying conditions .
Data Table: Applications Overview
Application Area | Specific Uses | Benefits |
---|---|---|
Polymer Chemistry | Cationic polymerization | High isotacticity, improved mechanical properties |
Medicinal Chemistry | Synthesis of pharmaceuticals | Formation of complex biologically active molecules |
Industrial Manufacturing | Adhesives, coatings, lubricants | Fast curing time, strong adhesion, weather resistance |
Case Study 1: Cationic Polymerization
Recent studies have demonstrated the effectiveness of this compound in cationic polymerization when used with specific initiators such as AgClO and diaryliodonium salts. These studies reveal that VIP can be polymerized under controlled conditions to yield materials with enhanced tensile strength and flexibility .
Case Study 2: Biologically Active Synthesis
Research has shown that this compound can be utilized to synthesize novel pharmaceutical compounds through multi-step reactions. One notable example involves the synthesis of anti-cancer agents where VIP was integrated into the molecular structure to enhance biological activity .
Mechanism of Action
The mechanism of action of propane, 2-(ethenyloxy)- involves its ability to undergo various chemical reactions due to the presence of the vinyl ether group. The vinyl group can participate in addition reactions, forming new bonds with other molecules. This reactivity makes it a valuable intermediate in organic synthesis .
Comparison with Similar Compounds
Physical and Thermal Properties
Key physical properties of VIPE and analogous vinyl ethers are compared below:
Key Findings :
- VIPE’s Tg (-3°C) is significantly higher than that of vinyl ethyl ether (-41°C) but lower than vinyl isobutyl ether (-19°C), making it suitable for moderately flexible polymers .
- Its boiling point (82–84°C) is intermediate among alkyl vinyl ethers, reflecting its branched structure’s impact on volatility .
Reactivity and Stability
- Thermal Decomposition: VIPE undergoes intramolecular decomposition at 447–521°C to form propylene and acetaldehyde, with an activation energy similar to vinyl ethyl ether. At 570°C, minor free radical decomposition occurs .
- Chemical Reactivity : VIPE participates in regioselective acylation reactions, as demonstrated in the synthesis of arbutin derivatives using immobilized enzymes. Its reactivity is comparable to vinyl isobutyl ether but slower than vinyl ethyl ether due to steric hindrance from the isopropyl group .
- Atmospheric Reactivity : Like other alkyl vinyl ethers, VIPE reacts with ozone to form secondary organic aerosols (SOA). However, its SOA yield is lower than that of linear analogs (e.g., n-butyl vinyl ether) due to reduced oxidative stability .
Biological Activity
Vinyl isopropyl ether (VIP) is a chemical compound with the molecular formula CHO and a molecular weight of 86.13 g/mol. It is classified as an ether and has various applications in organic synthesis and as a potential building block in polymer chemistry. This article explores the biological activity of this compound, focusing on its effects, mechanisms, and related research findings.
- IUPAC Name : 2-ethenoxypropane
- Synonyms : Isopropyl vinyl ether, Propane, 2-(ethenyloxy)-
- Molecular Weight : 86.13 g/mol
- Topological Polar Surface Area : 9.2 Ų
- Hydrogen Bond Acceptors : 1
1. Antioxidant Activity
Research indicates that compounds derived from vinyl ethers exhibit significant antioxidant properties. For instance, derivatives of phenolic acids, which can be synthesized using vinyl ethers as intermediates, have shown potent antioxidant activities. These properties are crucial for mitigating oxidative stress-related diseases.
2. Anti-inflammatory Effects
This compound's structural similarity to other bioactive compounds suggests it may possess anti-inflammatory properties. Compounds like methyl vanillate, derived from similar structures, have demonstrated anti-inflammatory effects in various studies .
3. Antimicrobial Properties
The antimicrobial activity of vinyl ether derivatives has been documented. Compounds such as arbutin esters synthesized from vinyl ethers have shown enhanced antimicrobial activity compared to their precursors . This suggests that VIP could potentially be used in formulations aimed at preventing microbial growth.
Case Study 1: Synthesis of Bioactive Esters
A study focused on the synthesis of aromatic esters from arbutin using vinyl vanillic acid and this compound as co-solvents. The results indicated that increasing the concentration of isopropyl ether up to 20% improved substrate conversion rates significantly, enhancing the overall yield of bioactive esters .
Table 1: Effect of Isopropyl Ether Concentration on Reaction Rates
Isopropyl Ether Concentration (%) | Reaction Rate (mM/h) | Substrate Conversion (%) |
---|---|---|
0 | 4.5 | 70 |
10 | 6.0 | 80 |
20 | 8.2 | 93 |
30 | 5.0 | 75 |
Case Study 2: Mechanistic Insights
Another research article investigated the enzymatic reaction mechanisms involving vinyl ethers in the synthesis of various bioactive compounds. The study highlighted how the presence of this compound influenced enzyme activity, suggesting a role in enhancing regioselectivity during acylation reactions .
Q & A
Basic Research Questions
Q. What are the established synthesis routes for vinyl isopropyl ether (VIPE), and how do reaction conditions influence yield?
VIPE is synthesized via Williamson ether synthesis or acid-catalyzed condensation. A common method involves reacting isopropyl alcohol with acetylene or vinyl n-butyl ether under controlled conditions. Catalysts like mercury and sodium acetates are critical for activating the vinyl group . Key parameters include temperature (optimal at the boiling point of the vinyl ether, ~68–70°C) and inert atmosphere to prevent side reactions. Yield optimization requires precise stoichiometric ratios (e.g., 1:1.2 alcohol-to-vinyl ether) and removal of water to shift equilibrium .
Q. What are the key physicochemical properties of VIPE relevant to laboratory handling?
VIPE (CAS 926-65-8) has a density of 0.7534 g/cm³, boiling point of ~68.84°C, and refractive index of 1.3840. Its low viscosity and high volatility necessitate storage in airtight containers at ≤4°C. The compound’s flammability (Hazard Class 3.1) and peroxide-forming tendency (via autoxidation) require inert gas purging and avoidance of light .
Q. How can researchers ensure purity and stability of VIPE during storage?
Use amber glass bottles under nitrogen or argon to suppress peroxide formation. Regular testing via iodometric titration or GC-MS is recommended. Add stabilizers like BHT (≤100 ppm) if prolonged storage is needed .
Advanced Research Questions
Q. What experimental designs address contradictions in ozonolysis studies of VIPE’s atmospheric reactivity?
Discrepancies in secondary organic aerosol (SOA) yields (e.g., 5–20% under varying NOx levels) arise from competing reaction pathways. Controlled experiments should:
- Standardize initial mixing ratios (e.g., 300 ppb VIPE).
- Use scavengers (e.g., cyclohexane) to isolate peroxy radical pathways.
- Employ mass spectrometry (CI-TOF) to track intermediates like Criegee radicals .
Q. How does VIPE’s structure influence its reactivity in living cationic polymerization?
The isopropoxy group stabilizes carbocations via electron donation, enabling controlled polymerization with initiating systems like HI/I₂. Kinetic studies show propagation rate constants (kₚ) of ~10³ L·mol⁻¹·s⁻¹ at 0°C. Adjusting solvent polarity (e.g., toluene vs. CH₂Cl₂) modulates chain-transfer rates and polydispersity (Đ < 1.2) .
Q. What analytical methods resolve discrepancies in VIPE’s reaction mechanisms with electrophiles?
Conflicting data on regioselectivity (e.g., Markovnikov vs. anti-Markovnikov addition) arise from solvent and catalyst effects. Use:
- In situ NMR to track intermediates.
- DFT calculations (B3LYP/6-311+G(d,p)) to model transition states.
- Isotopic labeling (²H/¹³C) to validate pathways .
Q. How can researchers design reproducible kinetic studies of VIPE’s hydrolysis?
Hydrolysis rates (pH-dependent) require:
- Buffered solutions (pH 2–12) with ionic strength control (I = 0.1 M KCl).
- Quenching with dry ice/acetone to arrest reactions.
- Headspace-free vials to prevent volatilization. Data normalization to reference compounds (e.g., ethyl vinyl ether) minimizes experimental error .
Q. Methodological Best Practices
Q. What statistical approaches validate VIPE’s structure-activity relationships (SAR) in toxicity studies?
Apply QSPR models using descriptors like logP (experimental: 1.2) and polar surface area (PSA: 9.2 Ų). Multivariate regression (e.g., PLS) identifies dominant factors (e.g., ether oxygen electronegativity) in cytotoxicity .
Q. How should researchers document VIPE-related data for reproducibility?
Follow AJEV guidelines:
Properties
IUPAC Name |
2-ethenoxypropane | |
---|---|---|
Source | PubChem | |
URL | https://pubchem.ncbi.nlm.nih.gov | |
Description | Data deposited in or computed by PubChem | |
InChI |
InChI=1S/C5H10O/c1-4-6-5(2)3/h4-5H,1H2,2-3H3 | |
Source | PubChem | |
URL | https://pubchem.ncbi.nlm.nih.gov | |
Description | Data deposited in or computed by PubChem | |
InChI Key |
GNUGVECARVKIPH-UHFFFAOYSA-N | |
Source | PubChem | |
URL | https://pubchem.ncbi.nlm.nih.gov | |
Description | Data deposited in or computed by PubChem | |
Canonical SMILES |
CC(C)OC=C | |
Source | PubChem | |
URL | https://pubchem.ncbi.nlm.nih.gov | |
Description | Data deposited in or computed by PubChem | |
Molecular Formula |
C5H10O | |
Source | PubChem | |
URL | https://pubchem.ncbi.nlm.nih.gov | |
Description | Data deposited in or computed by PubChem | |
Related CAS |
25585-49-3 | |
Record name | Propane, 2-(ethenyloxy)-, homopolymer | |
Source | CAS Common Chemistry | |
URL | https://commonchemistry.cas.org/detail?cas_rn=25585-49-3 | |
Description | CAS Common Chemistry is an open community resource for accessing chemical information. Nearly 500,000 chemical substances from CAS REGISTRY cover areas of community interest, including common and frequently regulated chemicals, and those relevant to high school and undergraduate chemistry classes. This chemical information, curated by our expert scientists, is provided in alignment with our mission as a division of the American Chemical Society. | |
Explanation | The data from CAS Common Chemistry is provided under a CC-BY-NC 4.0 license, unless otherwise stated. | |
DSSTOX Substance ID |
DTXSID0061292 | |
Record name | Propane, 2-(ethenyloxy)- | |
Source | EPA DSSTox | |
URL | https://comptox.epa.gov/dashboard/DTXSID0061292 | |
Description | DSSTox provides a high quality public chemistry resource for supporting improved predictive toxicology. | |
Molecular Weight |
86.13 g/mol | |
Source | PubChem | |
URL | https://pubchem.ncbi.nlm.nih.gov | |
Description | Data deposited in or computed by PubChem | |
CAS No. |
926-65-8 | |
Record name | Isopropyl vinyl ether | |
Source | CAS Common Chemistry | |
URL | https://commonchemistry.cas.org/detail?cas_rn=926-65-8 | |
Description | CAS Common Chemistry is an open community resource for accessing chemical information. Nearly 500,000 chemical substances from CAS REGISTRY cover areas of community interest, including common and frequently regulated chemicals, and those relevant to high school and undergraduate chemistry classes. This chemical information, curated by our expert scientists, is provided in alignment with our mission as a division of the American Chemical Society. | |
Explanation | The data from CAS Common Chemistry is provided under a CC-BY-NC 4.0 license, unless otherwise stated. | |
Record name | Propane, 2-(ethenyloxy)- | |
Source | ChemIDplus | |
URL | https://pubchem.ncbi.nlm.nih.gov/substance/?source=chemidplus&sourceid=0000926658 | |
Description | ChemIDplus is a free, web search system that provides access to the structure and nomenclature authority files used for the identification of chemical substances cited in National Library of Medicine (NLM) databases, including the TOXNET system. | |
Record name | Propane, 2-(ethenyloxy)- | |
Source | EPA Chemicals under the TSCA | |
URL | https://www.epa.gov/chemicals-under-tsca | |
Description | EPA Chemicals under the Toxic Substances Control Act (TSCA) collection contains information on chemicals and their regulations under TSCA, including non-confidential content from the TSCA Chemical Substance Inventory and Chemical Data Reporting. | |
Record name | Propane, 2-(ethenyloxy)- | |
Source | EPA DSSTox | |
URL | https://comptox.epa.gov/dashboard/DTXSID0061292 | |
Description | DSSTox provides a high quality public chemistry resource for supporting improved predictive toxicology. | |
Record name | 2-(vinyloxy)propane | |
Source | European Chemicals Agency (ECHA) | |
URL | https://echa.europa.eu/substance-information/-/substanceinfo/100.011.948 | |
Description | The European Chemicals Agency (ECHA) is an agency of the European Union which is the driving force among regulatory authorities in implementing the EU's groundbreaking chemicals legislation for the benefit of human health and the environment as well as for innovation and competitiveness. | |
Explanation | Use of the information, documents and data from the ECHA website is subject to the terms and conditions of this Legal Notice, and subject to other binding limitations provided for under applicable law, the information, documents and data made available on the ECHA website may be reproduced, distributed and/or used, totally or in part, for non-commercial purposes provided that ECHA is acknowledged as the source: "Source: European Chemicals Agency, http://echa.europa.eu/". Such acknowledgement must be included in each copy of the material. ECHA permits and encourages organisations and individuals to create links to the ECHA website under the following cumulative conditions: Links can only be made to webpages that provide a link to the Legal Notice page. | |
Retrosynthesis Analysis
AI-Powered Synthesis Planning: Our tool employs the Template_relevance Pistachio, Template_relevance Bkms_metabolic, Template_relevance Pistachio_ringbreaker, Template_relevance Reaxys, Template_relevance Reaxys_biocatalysis model, leveraging a vast database of chemical reactions to predict feasible synthetic routes.
One-Step Synthesis Focus: Specifically designed for one-step synthesis, it provides concise and direct routes for your target compounds, streamlining the synthesis process.
Accurate Predictions: Utilizing the extensive PISTACHIO, BKMS_METABOLIC, PISTACHIO_RINGBREAKER, REAXYS, REAXYS_BIOCATALYSIS database, our tool offers high-accuracy predictions, reflecting the latest in chemical research and data.
Strategy Settings
Precursor scoring | Relevance Heuristic |
---|---|
Min. plausibility | 0.01 |
Model | Template_relevance |
Template Set | Pistachio/Bkms_metabolic/Pistachio_ringbreaker/Reaxys/Reaxys_biocatalysis |
Top-N result to add to graph | 6 |
Feasible Synthetic Routes
Disclaimer and Information on In-Vitro Research Products
Please be aware that all articles and product information presented on BenchChem are intended solely for informational purposes. The products available for purchase on BenchChem are specifically designed for in-vitro studies, which are conducted outside of living organisms. In-vitro studies, derived from the Latin term "in glass," involve experiments performed in controlled laboratory settings using cells or tissues. It is important to note that these products are not categorized as medicines or drugs, and they have not received approval from the FDA for the prevention, treatment, or cure of any medical condition, ailment, or disease. We must emphasize that any form of bodily introduction of these products into humans or animals is strictly prohibited by law. It is essential to adhere to these guidelines to ensure compliance with legal and ethical standards in research and experimentation.