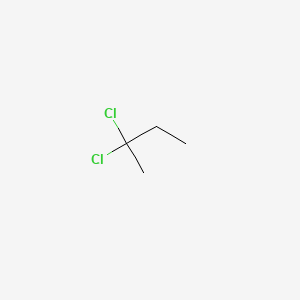
2,2-Dichlorobutane
Overview
Description
2,2-Dichlorobutane (CAS 4279-22-5) is a halogenated alkane with the molecular formula C₄H₈Cl₂ and a molecular weight of 127.01 g/mol . It exists as a colorless to yellowish transparent liquid with a boiling point of 104°C, density of 1.09 g/cm³, and refractive index of 1.43 . It is primarily used as a laboratory chemical and intermediate in organic synthesis .
Preparation Methods
Preparation from Butyne-(Z) and Hydrogen Chloride under Pressure
- The most efficient and industrially relevant method involves the addition of hydrogen chloride (HCl) to butyne-(Z) (2-butyne) under elevated pressure.
- Butyne-(Z) is introduced into an autoclave, and excess hydrogen chloride gas is forced in under pressures ranging from 1 to 50 atmospheres (preferably 20 to 40 atmospheres).
- The reaction temperature is maintained between -50°C and +100°C, with optimal yields achieved around 20 to 40°C with continuous cooling.
- The reaction progress is monitored by a decrease in pressure and an increase in temperature.
- Upon completion, excess HCl is vented, and the crude product is scrubbed with water and distilled to isolate 2,2-dichlorobutane.
Reaction Characteristics and Advantages:
- This noncatalytic process avoids the formation of monochlorinated by-products and is selective for this compound.
- The method yields approximately 60% of this compound based on butyne-(Z) input.
- The reaction avoids costly chlorinating agents like phosphorus pentachloride and minimizes isomeric by-products that complicate purification.
- The process is technically advantageous because butyne-(Z) is often a waste product in butadiene manufacture, providing a cost-effective feedstock.
Parameter | Value |
---|---|
Starting material | Butyne-(Z) |
Hydrogen chloride pressure | 30 atmospheres (gauge) |
Temperature | 20–40°C (reaction temperature) |
Reaction time | 0.5 to 1 hour |
Yield of this compound | ~60.5% (based on butyne-(Z)) |
Boiling point of product | 102–104°C |
Reference: Patent US3465054A details this process extensively, highlighting the reaction conditions and yields.
Chlorination of Butane or Butene Derivatives
- Traditional chlorination of butane, butene, or chlorobutane under light exposure produces this compound among other isomers.
- Chlorine gas is reacted with these hydrocarbons, often requiring catalysts and elevated temperatures.
- The reaction typically yields mixtures of chlorinated isomers, complicating purification by distillation.
- Yields of this compound are generally poor due to the formation of multiple isomeric chlorides.
- The presence of isomers impedes efficient separation.
- High temperatures and catalysts are often necessary, increasing process complexity.
Reference: The chlorination approach is mentioned in the context of by-product formation and is contrasted with the butyne-(Z) method.
Synthesis from Methylethylketone and Phosphorus Pentachloride
- This compound can be synthesized by chlorinating methylethylketone with phosphorus pentachloride (PCl5).
- This method involves converting the ketone functional group to the corresponding dichloride.
- The use of phosphorus pentachloride is costly and generates by-products that require careful handling.
- This method is less favored industrially compared to the butyne-(Z) route.
Reference: This method is noted as a known but less efficient approach in the patent literature.
Experimental Chlorination Using Sulfuryl Chloride
- Chlorination of 1-chlorobutane with sulfuryl chloride (SO2Cl2) under reflux conditions can produce various dichlorobutane isomers, including this compound.
- The reaction involves radical substitution mechanisms initiated by light or heat.
- The yield of dichlorobutane in such experiments can be low (~22.6%), partly due to losses during workup and separation.
Parameter | Value |
---|---|
Starting material | 1-Chlorobutane |
Chlorinating agent | Sulfuryl chloride (SO2Cl2) |
Yield of dichlorobutane | 22.6% (observed) |
Product mixture | Multiple dichlorobutane isomers |
Analysis method | Gas-Liquid Chromatography |
- Secondary hydrogens were found to be more reactive than primary hydrogens in substitution reactions, influencing isomer distribution.
Reference: Experimental data and analysis from an organic chemistry laboratory report.
Summary Table of Preparation Methods
Method | Starting Materials | Conditions | Yield (%) | Advantages | Limitations |
---|---|---|---|---|---|
Addition of HCl to Butyne-(Z) | Butyne-(Z), HCl | 20–40 atm, 20–40°C, 0.5–1 hr | ~60.5 | High selectivity, no catalysts | Requires high pressure equipment |
Chlorination of Butane/Butene | Butane, Butene, Cl2 | UV light, catalysts, elevated T | Low | Simple reagents | Low yield, isomer mixtures |
Chlorination of Methylethylketone | Methylethylketone, PCl5 | Ambient to elevated T | Not specified | Direct conversion from ketone | Costly reagents, by-products |
Sulfuryl Chloride Chlorination (Lab Scale) | 1-Chlorobutane, SO2Cl2 | Reflux, radical initiation | ~22.6 | Simple lab procedure | Low yield, mixture of isomers |
Chemical Reactions Analysis
2,2-Dichlorobutane undergoes various chemical reactions, including:
Substitution Reactions: In the presence of nucleophiles, this compound can undergo substitution reactions where the chlorine atoms are replaced by other functional groups.
Elimination Reactions: Under strong basic conditions, this compound can undergo elimination reactions to form alkenes.
Major Products Formed:
Substitution Products: Depending on the nucleophile used, the major products can include alcohols, ethers, or other substituted hydrocarbons.
Elimination Products: The major products of elimination reactions are butenes, such as 2-butene and 1-butene.
Scientific Research Applications
Chemical Synthesis
Intermediate in Organic Synthesis:
2,2-Dichlorobutane is widely used as an intermediate in the synthesis of pharmaceuticals and agrochemicals. Its chlorine substituents enhance electrophilicity, facilitating nucleophilic substitution reactions that are essential for creating complex organic molecules. For example, it can be utilized in the synthesis of chiral compounds, which are crucial in drug development.
Table 1: Examples of Compounds Synthesized Using this compound
Compound Type | Example Compound | Application Area |
---|---|---|
Pharmaceuticals | Chiral drugs | Medicine |
Agrochemicals | Herbicides | Agriculture |
Polymers | Poly(vinyl chloride) | Material science |
Material Science
Polymer Production:
In material science, this compound is used to produce polymers and other materials where halogenated hydrocarbons are required. The incorporation of chlorine atoms into polymer chains can enhance properties such as flame resistance and chemical stability.
Case Study: Modification of Aramid Fibers
Research has demonstrated that treating aramid fibers with 1,4-dichlorobutane can significantly improve their mechanical properties. In a study published in MDPI, aramid fibers modified with dichlorobutane showed increased tensile strength and interfacial shear strength when used in epoxy composites. The modification process involved supercritical CO2 treatment, which allowed for effective grafting of the dichlorobutane onto the fiber surface .
Degradation Studies:
The environmental impact of halogenated compounds like this compound is an area of active research. Studies focus on its degradation pathways and potential ecological effects when released into the environment. Understanding these pathways is crucial for developing strategies to mitigate pollution from industrial processes.
Mechanism of Action
The mechanism of action of 2,2-dichlorobutane in chemical reactions involves the interaction of its chlorine atoms with nucleophiles or bases. The chlorine atoms are electron-withdrawing groups, making the carbon atoms they are attached to more electrophilic. This electrophilicity facilitates nucleophilic substitution and elimination reactions. In substitution reactions, nucleophiles attack the electrophilic carbon, displacing the chlorine atoms. In elimination reactions, bases abstract a proton from the carbon adjacent to the chlorine, leading to the formation of a double bond and the release of hydrogen chloride .
Comparison with Similar Compounds
Comparison with Structural Isomers of Dichlorobutane
2,3-Dichlorobutane (CAS 7581-97-7)
- Molecular Formula : C₄H₈Cl₂ (same as 2,2-isomer) .
- Physical Properties: Limited data, but expected to have a lower boiling point than 2,2-dichlorobutane due to reduced molecular symmetry .
- Stereoisomerism : Exhibits optical isomerism (two enantiomers: R,R and S,S) and a meso form (R,S) due to an internal plane of symmetry . This contrasts with this compound, which lacks stereoisomerism .
- Applications : Used in academic studies of stereochemistry and reaction mechanisms .
1,2-Dichlorobutane and 1,3-Dichlorobutane
- 1,2-Dichlorobutane: Produced via radical chlorination of 1-chlorobutane .
- 1,3-Dichlorobutane: Limited industrial use; safety data highlights acute toxicity risks (H302) .
1,4-Dichlorobutane
- Applications :
Comparison with Other Halogenated Compounds
2,2-Dichloro-3,3-dimethylbutane
- Molecular Formula : C₆H₁₂Cl₂ (larger branched structure) .
- Physical Properties : Higher molecular weight (155.06 g/mol) and boiling point compared to this compound .
- Applications : Used in specialty organic syntheses due to steric hindrance from methyl groups .
Haloacids and Haloketones
- 2,2-Dichlorobutanoic Acid (CAS 13023-00-2): A haloacid with marine toxicity (Mar classification) .
- 1,1-Dichloro-2-butanone (CAS 2648-56-8): A haloketone with moderate carcinogenic potency (LM classification) .
Data Tables
Table 1: Physical Properties of Dichlorobutane Isomers
Compound | CAS Number | Boiling Point (°C) | Density (g/cm³) | Molecular Weight (g/mol) |
---|---|---|---|---|
This compound | 4279-22-5 | 104 | 1.09 | 127.01 |
2,3-Dichlorobutane | 7581-97-7 | ~95 (estimated) | ~1.10 | 127.01 |
1,4-Dichlorobutane | Not provided | Not reported | Not reported | 127.01 |
Biological Activity
2,2-Dichlorobutane is a chlorinated hydrocarbon with significant implications in both industrial applications and biological research. Its chemical structure, characterized by two chlorine atoms attached to the second carbon of a four-carbon butane chain, positions it as a compound of interest for studying mutagenicity, genotoxicity, and other biological activities.
- Chemical Formula : C4H8Cl2
- Molecular Weight : 147.01 g/mol
- Boiling Point : Approximately 88 °C
Biological Activity Overview
Research has indicated that this compound exhibits various biological activities, particularly concerning its mutagenic and genotoxic properties. The following sections detail findings from studies that highlight its effects on cellular mechanisms.
Mutagenicity and Genotoxicity
A significant study evaluated the mutagenicity and genotoxicity of several chlorinated hydrocarbons, including this compound. The micronucleus (MN) test and the alkaline single-cell gel electrophoresis (Comet) assay were employed to assess potential DNA damage:
- Findings :
- Mutagenic Activity : this compound did not show significant mutagenic activity in the MN test across various concentrations tested .
- Genotoxic Effects : Although not directly mutagenic, it was noted that other chlorinated compounds exhibited strong DNA damage effects, suggesting a complex interaction where this compound may play a role in the presence of other agents .
Study on Chlorinated Hydrocarbons
A comprehensive analysis involving multiple chlorinated hydrocarbons showed that while this compound itself did not induce clear mutagenic effects, it was part of a broader study that assessed its behavior alongside more potent mutagens. This study utilized human lymphocytes to evaluate cytotoxicity and DNA breakage capacity .
QSAR Analysis
Quantitative Structure-Activity Relationship (QSAR) models were developed based on the toxicity data from various chlorinated compounds. The analysis indicated that the toxicity was influenced by factors such as lipophilicity and molecular structure. While this compound was not highlighted as a primary concern, its structural characteristics were included in broader assessments of related compounds .
Biological Activity Table
Synthesis and Related Compounds
Recent studies have synthesized derivatives of this compound for various applications. For instance, amide-type compounds derived from this base compound demonstrated moderate fungicidal and insecticidal activities. These derivatives are being explored for their potential agricultural applications .
Q & A
Basic Questions
Q. Q1. What experimental methods are recommended to verify the purity and structural identity of 2,2-dichlorobutane in laboratory settings?
To confirm purity, use gas chromatography (GC) with flame ionization detection, as retention times and peak areas correlate with compound abundance and identity. For structural verification, employ nuclear magnetic resonance (NMR) spectroscopy (¹H and ¹³C) to analyze the chemical environment of the two equivalent chlorine atoms on the C2 carbon. Cross-reference with infrared (IR) spectroscopy to detect characteristic C-Cl stretching vibrations (~550–850 cm⁻¹). Density (1.09 g/cm³) and refractive index (1.428–1.432) can serve as supplementary purity indicators .
Q. Q2. How does the absence of optical isomerism in this compound influence its stereochemical applications compared to other dichlorobutane isomers?
this compound lacks chiral centers due to the two chlorine atoms being on the same carbon (C2), resulting in a plane of symmetry. This distinguishes it from 2,3-dichlorobutane, which has two chiral centers and exhibits optical isomerism. Researchers must account for this when designing stereoselective reactions, as this compound cannot form enantiomers, simplifying chiral separation steps in synthetic pathways .
Intermediate Questions
Q. Q3. How can discrepancies between theoretical and experimental isomer ratios in radical halogenation reactions involving this compound be resolved?
In radical chlorination of butane, the expected ratio of this compound depends on the relative stability of secondary radicals. However, experimental GC data often show deviations (e.g., 5% observed vs. 16.7% predicted for 1,1-dichlorobutane). To resolve this, analyze reaction conditions (e.g., temperature, initiator concentration) and steric effects. Computational modeling of transition states using software like Gaussian can provide mechanistic insights into selectivity .
Q. Q4. What safety protocols are critical when handling this compound in high-temperature reactions?
Due to its low flash point (27°C) and flammability, use inert atmospheres (N₂/Ar) and explosion-proof equipment. Implement secondary containment for spills and avoid contact with oxidizers. Personal protective equipment (PPE) must include nitrile gloves, goggles, and fume hoods. Toxicity data gaps (e.g., carcinogenicity) necessitate adherence to ALARA (As Low As Reasonably Achievable) exposure principles .
Advanced Questions
Q. Q5. How can computational chemistry predict the thermodynamic stability and reaction pathways of this compound?
Density functional theory (DFT) calculations (e.g., B3LYP/6-31G*) can optimize the molecular geometry and calculate Gibbs free energy for decomposition products (e.g., HCl, butenes). Use the NIST Chemistry WebBook for thermochemical data (e.g., enthalpy of formation). Molecular dynamics simulations can model solvent interactions, aiding in solvent selection for synthesis or extraction .
Q. Q6. What analytical challenges arise when quantifying trace this compound in environmental samples, and how can they be mitigated?
Low water solubility and high volatility complicate detection in aqueous matrices. Use purge-and-trap GC-MS with electron capture detection (ECD) for ppb-level sensitivity. For soil/sediment, employ Soxhlet extraction with dichloromethane. Matrix effects can be minimized via isotope dilution (e.g., ²H-labeled analogs) .
Q. Research Design & Data Interpretation
Q. Q7. How would you design an experiment to study the kinetic vs. thermodynamic control in the synthesis of this compound?
Vary reaction temperature and monitor product ratios via GC. At low temperatures (kinetic control), the less stable but faster-forming isomer dominates. At high temperatures (thermodynamic control), the more stable isomer prevails. Compare activation energies (Eₐ) via Arrhenius plots and compute transition-state energies using computational tools .
Q. Q8. What mechanistic insights can be drawn from the regioselectivity of this compound formation in electrophilic addition reactions?
In allylic chlorination, the preference for this compound over 1,2-isomers suggests a radical stabilization mechanism. Electron paramagnetic resonance (EPR) can detect radical intermediates. Compare with Markovnikov addition trends to distinguish between ionic and radical pathways .
Q. Methodological Gaps & Future Directions
Q. Q9. Why is there a lack of ecotoxicological data for this compound, and how can researchers address this?
Existing studies focus on acute toxicity (e.g., LC₅₀ for fish), but chronic and genotoxic effects are poorly documented. Propose tiered testing: (1) in vitro Ames tests for mutagenicity, (2) Daphnia magna chronic exposure assays, and (3) soil microcosm studies to assess biodegradation pathways .
Q. Q10. How can advanced material science techniques (e.g., XRD, AFM) elucidate the role of this compound in polymer modification?
X-ray diffraction (XRD) can measure crystallinity changes in polymers treated with this compound. Atomic force microscopy (AFM) can map surface topography modifications. Compare with 1,4-dichlorobutane-treated samples to study the impact of chlorine positioning on material properties .
Properties
IUPAC Name |
2,2-dichlorobutane | |
---|---|---|
Source | PubChem | |
URL | https://pubchem.ncbi.nlm.nih.gov | |
Description | Data deposited in or computed by PubChem | |
InChI |
InChI=1S/C4H8Cl2/c1-3-4(2,5)6/h3H2,1-2H3 | |
Source | PubChem | |
URL | https://pubchem.ncbi.nlm.nih.gov | |
Description | Data deposited in or computed by PubChem | |
InChI Key |
BSRTYNDWQXVCKR-UHFFFAOYSA-N | |
Source | PubChem | |
URL | https://pubchem.ncbi.nlm.nih.gov | |
Description | Data deposited in or computed by PubChem | |
Canonical SMILES |
CCC(C)(Cl)Cl | |
Source | PubChem | |
URL | https://pubchem.ncbi.nlm.nih.gov | |
Description | Data deposited in or computed by PubChem | |
Molecular Formula |
C4H8Cl2 | |
Source | PubChem | |
URL | https://pubchem.ncbi.nlm.nih.gov | |
Description | Data deposited in or computed by PubChem | |
DSSTOX Substance ID |
DTXSID2063391 | |
Record name | Butane, 2,2-dichloro- | |
Source | EPA DSSTox | |
URL | https://comptox.epa.gov/dashboard/DTXSID2063391 | |
Description | DSSTox provides a high quality public chemistry resource for supporting improved predictive toxicology. | |
Molecular Weight |
127.01 g/mol | |
Source | PubChem | |
URL | https://pubchem.ncbi.nlm.nih.gov | |
Description | Data deposited in or computed by PubChem | |
CAS No. |
4279-22-5 | |
Record name | 2,2-Dichlorobutane | |
Source | CAS Common Chemistry | |
URL | https://commonchemistry.cas.org/detail?cas_rn=4279-22-5 | |
Description | CAS Common Chemistry is an open community resource for accessing chemical information. Nearly 500,000 chemical substances from CAS REGISTRY cover areas of community interest, including common and frequently regulated chemicals, and those relevant to high school and undergraduate chemistry classes. This chemical information, curated by our expert scientists, is provided in alignment with our mission as a division of the American Chemical Society. | |
Explanation | The data from CAS Common Chemistry is provided under a CC-BY-NC 4.0 license, unless otherwise stated. | |
Record name | Butane, 2,2-dichloro- | |
Source | ChemIDplus | |
URL | https://pubchem.ncbi.nlm.nih.gov/substance/?source=chemidplus&sourceid=0004279225 | |
Description | ChemIDplus is a free, web search system that provides access to the structure and nomenclature authority files used for the identification of chemical substances cited in National Library of Medicine (NLM) databases, including the TOXNET system. | |
Record name | Butane, 2,2-dichloro- | |
Source | EPA Chemicals under the TSCA | |
URL | https://www.epa.gov/chemicals-under-tsca | |
Description | EPA Chemicals under the Toxic Substances Control Act (TSCA) collection contains information on chemicals and their regulations under TSCA, including non-confidential content from the TSCA Chemical Substance Inventory and Chemical Data Reporting. | |
Record name | Butane, 2,2-dichloro- | |
Source | EPA DSSTox | |
URL | https://comptox.epa.gov/dashboard/DTXSID2063391 | |
Description | DSSTox provides a high quality public chemistry resource for supporting improved predictive toxicology. | |
Record name | 2,2-dichlorobutane | |
Source | European Chemicals Agency (ECHA) | |
URL | https://echa.europa.eu/substance-information/-/substanceinfo/100.022.076 | |
Description | The European Chemicals Agency (ECHA) is an agency of the European Union which is the driving force among regulatory authorities in implementing the EU's groundbreaking chemicals legislation for the benefit of human health and the environment as well as for innovation and competitiveness. | |
Explanation | Use of the information, documents and data from the ECHA website is subject to the terms and conditions of this Legal Notice, and subject to other binding limitations provided for under applicable law, the information, documents and data made available on the ECHA website may be reproduced, distributed and/or used, totally or in part, for non-commercial purposes provided that ECHA is acknowledged as the source: "Source: European Chemicals Agency, http://echa.europa.eu/". Such acknowledgement must be included in each copy of the material. ECHA permits and encourages organisations and individuals to create links to the ECHA website under the following cumulative conditions: Links can only be made to webpages that provide a link to the Legal Notice page. | |
Retrosynthesis Analysis
AI-Powered Synthesis Planning: Our tool employs the Template_relevance Pistachio, Template_relevance Bkms_metabolic, Template_relevance Pistachio_ringbreaker, Template_relevance Reaxys, Template_relevance Reaxys_biocatalysis model, leveraging a vast database of chemical reactions to predict feasible synthetic routes.
One-Step Synthesis Focus: Specifically designed for one-step synthesis, it provides concise and direct routes for your target compounds, streamlining the synthesis process.
Accurate Predictions: Utilizing the extensive PISTACHIO, BKMS_METABOLIC, PISTACHIO_RINGBREAKER, REAXYS, REAXYS_BIOCATALYSIS database, our tool offers high-accuracy predictions, reflecting the latest in chemical research and data.
Strategy Settings
Precursor scoring | Relevance Heuristic |
---|---|
Min. plausibility | 0.01 |
Model | Template_relevance |
Template Set | Pistachio/Bkms_metabolic/Pistachio_ringbreaker/Reaxys/Reaxys_biocatalysis |
Top-N result to add to graph | 6 |
Feasible Synthetic Routes
Disclaimer and Information on In-Vitro Research Products
Please be aware that all articles and product information presented on BenchChem are intended solely for informational purposes. The products available for purchase on BenchChem are specifically designed for in-vitro studies, which are conducted outside of living organisms. In-vitro studies, derived from the Latin term "in glass," involve experiments performed in controlled laboratory settings using cells or tissues. It is important to note that these products are not categorized as medicines or drugs, and they have not received approval from the FDA for the prevention, treatment, or cure of any medical condition, ailment, or disease. We must emphasize that any form of bodily introduction of these products into humans or animals is strictly prohibited by law. It is essential to adhere to these guidelines to ensure compliance with legal and ethical standards in research and experimentation.