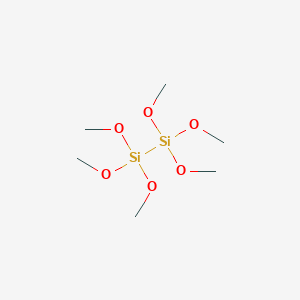
Hexamethoxydisilane
Overview
Description
Hexamethoxydisilane is an organosilicon compound with the molecular formula ( \text{C}6\text{H}{18}\text{O}_6\text{Si}_2 ). It is a colorless liquid that is primarily used as a precursor in the synthesis of other silicon-containing compounds. This compound is known for its high reactivity and versatility in various chemical reactions, making it a valuable reagent in both academic and industrial settings.
Preparation Methods
Synthetic Routes and Reaction Conditions: Hexamethoxydisilane can be synthesized through the reaction of silicon tetrachloride with methanol in the presence of a base such as pyridine. The reaction proceeds as follows: [ \text{SiCl}_4 + 6 \text{CH}_3\text{OH} \rightarrow \text{Si(OCH}_3)_4 + 4 \text{HCl} ] This reaction typically requires anhydrous conditions and is carried out at low temperatures to prevent the formation of unwanted by-products.
Industrial Production Methods: In an industrial setting, this compound is produced using a continuous flow reactor to ensure consistent quality and yield. The process involves the controlled addition of silicon tetrachloride and methanol, followed by the removal of hydrogen chloride gas. The product is then purified through distillation to obtain high-purity this compound.
Chemical Reactions Analysis
Types of Reactions: Hexamethoxydisilane undergoes various types of chemical reactions, including:
Hydrolysis: Reacts with water to form silicic acid and methanol.
Condensation: Forms siloxane bonds when reacted with other silanes or silanols.
Substitution: Can undergo nucleophilic substitution reactions with halides or other nucleophiles.
Common Reagents and Conditions:
Hydrolysis: Typically carried out in the presence of a catalyst such as hydrochloric acid or sulfuric acid.
Condensation: Often performed under anhydrous conditions using catalysts like tin or titanium compounds.
Substitution: Requires the presence of a nucleophile such as an alcohol or amine.
Major Products Formed:
Hydrolysis: Produces silicic acid and methanol.
Condensation: Forms siloxane polymers or oligomers.
Substitution: Yields various organosilicon compounds depending on the nucleophile used.
Scientific Research Applications
Chemical Synthesis
1.1. Coupling Agent in Organic Reactions
HMDS is employed as a coupling agent in the formation of amides from carboxylic acids and amines. A solvent-free procedure has been developed for this purpose, demonstrating good to excellent yields of amides when using HMDS as a coupling agent. This method showcases the compound's efficiency in facilitating peptide bond formation, surpassing traditional monosilanes in terms of yield and group tolerance .
1.2. Silica-Based Materials
The sol-gel process involving HMDS is fundamental in synthesizing silica-based materials with luminescent properties. Research indicates that HMDS contributes to the formation of silica networks that exhibit varied luminescence depending on the processing conditions. The photoluminescence (PL) spectra analysis reveals that samples prepared with HMDS show distinct luminescent behaviors, making them suitable for optoelectronic applications .
Nanotechnology Applications
2.1. Coating and Surface Modification
HMDS is frequently used in the fabrication of thin films and coatings for semiconductor devices. Its ability to form stable siloxane networks allows for enhanced surface properties such as hydrophobicity and chemical resistance. These coatings are critical in improving device performance and longevity, particularly in microelectronics .
2.2. Precursor for Nanostructured Silicon
As a precursor for silicon nanostructures, HMDS can be thermally decomposed to produce silicon nanoparticles or films. This application is significant in developing photovoltaic cells and other electronic components where silicon plays a crucial role .
Biomedical Applications
3.1. Drug Delivery Systems
Recent studies have explored the use of HMDS in drug delivery systems, particularly as a component in biodegradable polymers that can effectively encapsulate therapeutic agents. The incorporation of HMDS enhances the mechanical properties of these polymers, allowing for controlled release profiles that are beneficial for targeted therapies .
3.2. Biocompatible Materials
The modification of biomaterials with HMDS has shown promise in enhancing biocompatibility and reducing protein adsorption, which is essential for implants and medical devices . This application highlights the compound's potential in improving interactions between synthetic materials and biological tissues.
Data Tables
Application Area | Description | Key Benefits |
---|---|---|
Chemical Synthesis | Coupling agent for amide formation | High yields, solvent-free process |
Nanotechnology | Coatings for semiconductors | Enhanced surface properties |
Nanostructured Silicon | Precursor for silicon nanoparticles | Essential for electronic components |
Biomedical Applications | Component in drug delivery systems | Controlled release profiles |
Biocompatible Materials | Modification to enhance biocompatibility | Improved tissue interaction |
Case Studies
Case Study 1: Amide Bond Formation
A study conducted on the use of HMDS as a coupling agent demonstrated that it could successfully facilitate the formation of amides from various carboxylic acids and amines under solvent-free conditions, achieving yields above 90%. The reaction was monitored using NMR spectroscopy, confirming the efficacy of HMDS in organic synthesis .
Case Study 2: Luminescent Silica Materials
Research involving sol-gel synthesis with HMDS revealed that varying annealing temperatures significantly affected the luminescent properties of silica-based materials. The study indicated optimal conditions where samples exhibited strong photoluminescence, suggesting potential applications in optoelectronics .
Mechanism of Action
The mechanism by which hexamethoxydisilane exerts its effects involves the formation of siloxane bonds through hydrolysis and condensation reactions. The compound’s high reactivity allows it to readily form bonds with other silicon-containing compounds, leading to the creation of complex silicon-based materials. The molecular targets and pathways involved in these reactions include the activation of silicon-oxygen bonds and the formation of stable siloxane linkages.
Comparison with Similar Compounds
Hexamethoxydisilane is unique in its high reactivity and versatility compared to other similar compounds. Some similar compounds include:
Tetramethoxysilane: Less reactive and primarily used in the production of silica.
Hexamethyldisilazane: Used as a silylation agent and has different reactivity due to the presence of nitrogen.
Trimethoxysilane: Similar in reactivity but used in different applications such as surface modification.
This compound stands out due to its ability to form a wide range of silicon-based materials and its applicability in various fields, from chemistry to medicine.
Biological Activity
Hexamethoxydisilane (HMDS) is a silane compound with significant implications in various fields, including materials science and biomedicine. This article explores the biological activity of HMDS, focusing on its pharmacological properties, potential therapeutic applications, and the mechanisms underlying its biological effects.
Overview of this compound
This compound is a silane compound with the chemical formula . It is primarily utilized as a coupling agent in polymer chemistry and as a precursor in the synthesis of silica-based materials. Recent studies have indicated that HMDS may also exhibit biological activities that warrant further investigation.
Pharmacological Properties
Research into the biological activity of HMDS is still emerging, but several studies have highlighted its potential pharmacological effects:
- Antimicrobial Activity : Preliminary studies suggest that HMDS may possess antimicrobial properties. For example, it has been evaluated for its effectiveness against various bacterial strains, showing potential as a disinfectant in clinical settings.
- Anti-inflammatory Effects : Some investigations have indicated that HMDS may exert anti-inflammatory effects. In vitro assays have demonstrated its ability to modulate inflammatory pathways, which could be beneficial in treating conditions characterized by excessive inflammation.
The biological activity of HMDS can be attributed to several mechanisms:
- Cell Membrane Interaction : HMDS may interact with cell membranes, affecting membrane fluidity and permeability. This interaction can disrupt microbial membranes, leading to cell lysis.
- Reactive Oxygen Species (ROS) Modulation : There is evidence suggesting that HMDS can influence ROS levels within cells, potentially leading to oxidative stress or cellular signaling changes that affect inflammation and cell survival.
Case Studies and Research Findings
Several case studies have been conducted to evaluate the biological activity of HMDS:
-
Antimicrobial Efficacy Study :
- Objective : To assess the antimicrobial properties of HMDS against Gram-positive and Gram-negative bacteria.
- Methodology : Disk diffusion method was employed to determine the inhibition zones around disks impregnated with HMDS.
- Results : Significant inhibition was observed against Staphylococcus aureus and Escherichia coli, indicating its potential as an antimicrobial agent.
-
In vitro Anti-inflammatory Study :
- Objective : To evaluate the anti-inflammatory effects of HMDS on cultured macrophages.
- Methodology : Macrophages were treated with HMDS and stimulated with lipopolysaccharides (LPS) to induce inflammation. Cytokine levels were measured using ELISA.
- Results : A marked reduction in pro-inflammatory cytokines (e.g., TNF-α, IL-6) was noted, suggesting that HMDS may inhibit inflammatory responses.
Data Table: Summary of Biological Activities
Q & A
Basic Research Questions
Q. What are the foundational physicochemical properties of hexamethoxydisilane, and how are they characterized in synthetic chemistry?
this compound’s properties, such as volatility, moisture sensitivity, and Si–Si bond reactivity, are critical for its use as a coupling agent. Characterization typically involves nuclear magnetic resonance (NMR) spectroscopy for structural analysis, gas chromatography-mass spectrometry (GC-MS) for purity assessment, and thermogravimetric analysis (TGA) for thermal stability. Experimental protocols should specify inert atmosphere conditions (e.g., nitrogen/argon) to prevent hydrolysis during handling .
Q. How do researchers optimize reaction conditions for this compound-mediated amide bond formation?
Optimization involves systematic variation of parameters such as stoichiometry (acid:amine:silane ratio), temperature, and reaction time. Design of Experiments (DOE) methodologies are recommended to identify optimal conditions. For example, benchmark studies using benzoic acid and benzylamine under solvent-free conditions can establish baseline efficiency, with methanol release monitored via GC .
Q. What safety protocols are essential when handling this compound in laboratory settings?
Due to its moisture sensitivity and potential exothermic reactivity, this compound requires storage under inert conditions and use in sealed systems (e.g., GC vials with pressure-release needles). Safety data sheets (SDS) and institutional guidelines for silane compounds must be followed, including fume hood use and emergency neutralization protocols for accidental exposure .
Advanced Research Questions
Q. How can contradictions in reported catalytic efficiencies of this compound across studies be resolved?
Discrepancies may arise from differences in reactant purity, moisture levels, or analytical techniques. Researchers should perform sensitivity analyses using controlled replicates and validate results with orthogonal methods (e.g., comparing NMR yields with gravimetric measurements). Contradiction frameworks, such as empirical falsification protocols, can isolate variables affecting reproducibility .
Q. What mechanistic insights explain the role of Si–Si bonds in this compound’s reactivity?
Advanced studies combine kinetic profiling (e.g., in-situ Fourier-transform infrared spectroscopy, FTIR) with computational modeling (density functional theory, DFT) to elucidate reaction pathways. The lower bond energy of Si–Si bonds compared to Si–O bonds facilitates cleavage under mild conditions, enabling nucleophilic attack by carboxylic acids or amines. Isotopic labeling experiments (e.g., deuterated substrates) can track intermediate formation .
Q. How do multivariate interactions (e.g., solvent, temperature, and catalyst) influence this compound’s performance in peptide synthesis?
Multivariate analysis via response surface methodology (RSM) identifies synergistic or antagonistic effects. For instance, solvent-free conditions may enhance reaction rates but require precise temperature control to avoid side reactions. Advanced DOE tools (e.g., partial least squares regression) quantify parameter contributions to yield and selectivity .
Q. What strategies address reproducibility challenges in this compound-based methodologies?
Reproducibility requires rigorous documentation of raw data (e.g., NMR spectra, chromatograms) and metadata (e.g., humidity levels, equipment calibration). Peer validation through inter-laboratory studies or open-source data sharing platforms (e.g., Zenodo) enhances reliability. Protocols should include step-by-step video demonstrations for critical steps like silane handling .
Q. Data Analysis and Contradiction Management
Q. How should researchers analyze discrepancies in thermal stability data for this compound?
Conflicting TGA/DSC results may stem from sample degradation during analysis or instrumental variability. Cross-validation with dynamic mechanical analysis (DMA) or isothermal calorimetry is advised. Statistical tools like Grubbs’ test can identify outliers, while error propagation models quantify uncertainty in decomposition temperatures .
Q. What frameworks guide the formulation of hypothesis-driven research questions for this compound studies?
The FINER (Feasible, Interesting, Novel, Ethical, Relevant) criteria ensure questions address knowledge gaps (e.g., "How does this compound compare to tetraalkoxysilanes in stereoselective synthesis?"). PICO (Population, Intervention, Comparison, Outcome) frameworks structure comparative studies, such as evaluating silane efficiency across substrate classes .
Q. Methodological Best Practices
Q. How should synthesis protocols using this compound be documented for peer review?
Reports must detail reaction vessels (e.g., GC vials with pressure-release mechanisms), purification methods (e.g., distillation under reduced pressure), and yield calculation formulas. Raw data (e.g., GC-MS traces) should be archived in appendices, with processed data (normalized yields, error margins) in the main text .
Q. What statistical approaches validate the significance of this compound’s catalytic effects?
Use ANOVA to compare yields across experimental groups, supplemented by post-hoc tests (e.g., Tukey’s HSD). For small datasets, non-parametric tests (Mann-Whitney U) are appropriate. Effect size metrics (Cohen’s d) quantify practical significance beyond p-values .
Properties
IUPAC Name |
trimethoxy(trimethoxysilyl)silane | |
---|---|---|
Source | PubChem | |
URL | https://pubchem.ncbi.nlm.nih.gov | |
Description | Data deposited in or computed by PubChem | |
InChI |
InChI=1S/C6H18O6Si2/c1-7-13(8-2,9-3)14(10-4,11-5)12-6/h1-6H3 | |
Source | PubChem | |
URL | https://pubchem.ncbi.nlm.nih.gov | |
Description | Data deposited in or computed by PubChem | |
InChI Key |
LMQGXNPPTQOGDG-UHFFFAOYSA-N | |
Source | PubChem | |
URL | https://pubchem.ncbi.nlm.nih.gov | |
Description | Data deposited in or computed by PubChem | |
Canonical SMILES |
CO[Si](OC)(OC)[Si](OC)(OC)OC | |
Source | PubChem | |
URL | https://pubchem.ncbi.nlm.nih.gov | |
Description | Data deposited in or computed by PubChem | |
Molecular Formula |
C6H18O6Si2 | |
Source | PubChem | |
URL | https://pubchem.ncbi.nlm.nih.gov | |
Description | Data deposited in or computed by PubChem | |
DSSTOX Substance ID |
DTXSID80345845 | |
Record name | Hexamethoxydisilane | |
Source | EPA DSSTox | |
URL | https://comptox.epa.gov/dashboard/DTXSID80345845 | |
Description | DSSTox provides a high quality public chemistry resource for supporting improved predictive toxicology. | |
Molecular Weight |
242.37 g/mol | |
Source | PubChem | |
URL | https://pubchem.ncbi.nlm.nih.gov | |
Description | Data deposited in or computed by PubChem | |
CAS No. |
5851-07-0 | |
Record name | Hexamethoxydisilane | |
Source | EPA DSSTox | |
URL | https://comptox.epa.gov/dashboard/DTXSID80345845 | |
Description | DSSTox provides a high quality public chemistry resource for supporting improved predictive toxicology. | |
Record name | Hexamethoxydisilane | |
Source | European Chemicals Agency (ECHA) | |
URL | https://echa.europa.eu/information-on-chemicals | |
Description | The European Chemicals Agency (ECHA) is an agency of the European Union which is the driving force among regulatory authorities in implementing the EU's groundbreaking chemicals legislation for the benefit of human health and the environment as well as for innovation and competitiveness. | |
Explanation | Use of the information, documents and data from the ECHA website is subject to the terms and conditions of this Legal Notice, and subject to other binding limitations provided for under applicable law, the information, documents and data made available on the ECHA website may be reproduced, distributed and/or used, totally or in part, for non-commercial purposes provided that ECHA is acknowledged as the source: "Source: European Chemicals Agency, http://echa.europa.eu/". Such acknowledgement must be included in each copy of the material. ECHA permits and encourages organisations and individuals to create links to the ECHA website under the following cumulative conditions: Links can only be made to webpages that provide a link to the Legal Notice page. | |
Retrosynthesis Analysis
AI-Powered Synthesis Planning: Our tool employs the Template_relevance Pistachio, Template_relevance Bkms_metabolic, Template_relevance Pistachio_ringbreaker, Template_relevance Reaxys, Template_relevance Reaxys_biocatalysis model, leveraging a vast database of chemical reactions to predict feasible synthetic routes.
One-Step Synthesis Focus: Specifically designed for one-step synthesis, it provides concise and direct routes for your target compounds, streamlining the synthesis process.
Accurate Predictions: Utilizing the extensive PISTACHIO, BKMS_METABOLIC, PISTACHIO_RINGBREAKER, REAXYS, REAXYS_BIOCATALYSIS database, our tool offers high-accuracy predictions, reflecting the latest in chemical research and data.
Strategy Settings
Precursor scoring | Relevance Heuristic |
---|---|
Min. plausibility | 0.01 |
Model | Template_relevance |
Template Set | Pistachio/Bkms_metabolic/Pistachio_ringbreaker/Reaxys/Reaxys_biocatalysis |
Top-N result to add to graph | 6 |
Feasible Synthetic Routes
Disclaimer and Information on In-Vitro Research Products
Please be aware that all articles and product information presented on BenchChem are intended solely for informational purposes. The products available for purchase on BenchChem are specifically designed for in-vitro studies, which are conducted outside of living organisms. In-vitro studies, derived from the Latin term "in glass," involve experiments performed in controlled laboratory settings using cells or tissues. It is important to note that these products are not categorized as medicines or drugs, and they have not received approval from the FDA for the prevention, treatment, or cure of any medical condition, ailment, or disease. We must emphasize that any form of bodily introduction of these products into humans or animals is strictly prohibited by law. It is essential to adhere to these guidelines to ensure compliance with legal and ethical standards in research and experimentation.