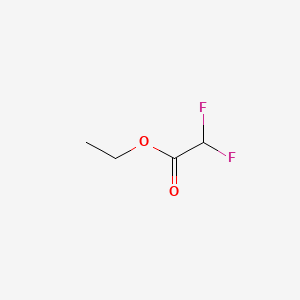
Ethyl difluoroacetate
Overview
Description
Ethyl difluoroacetate is used in pharmaceutical intermediates, acrylization oxidation, and halogenating reaction of catalyst . It is also used in preparing pyrrazolo-pyrimidine derivatives .
Synthesis Analysis
This compound can be synthesized through various methods. One method involves the use of ethanol, N,N-dimethyl acetamide, and difluoroacetic acid fluoride . The reaction liquid is distilled under reduced pressure to extract this compound .
Molecular Structure Analysis
The molecular formula of this compound is C4H6F2O2 . Its molecular weight is 124.09 .
Chemical Reactions Analysis
This compound can be degraded to difluoroacetic acid by strains of Actinobacteria . When introduced into a reaction, it can affect the cycle performance of the battery .
Physical and Chemical Properties Analysis
This compound is a clear colorless liquid . It has a refractive index of 1.347 and a density of 1.18 g/mL at 25 °C .
Scientific Research Applications
Radical Reactions and Synthesis of Organic Compounds
- Synthesis of 3,3-Difluoro-GABA: Ethyl/methyl 2-bromo-2,2-difluoroacetate is used in radical additions to vinyl ethers, forming difluoroacetyl-substituted acetals. This method was key in synthesizing 3,3-difluoro-GABA, an isomer of difluoro GABAs (Kondratov et al., 2015).
- Electrochemical Reduction: The electrochemical behavior of difluoroacetic ethyl ester has been studied, highlighting its reduction at a mercury cathode and the cleavage of the C-F bond, contributing to the understanding of its electrochemical properties (Inesi & Rampazzo, 1974).
- As a Building Block Precursor: Ethyl-2,2-difluoro-2-trimethylsilylacetate, prepared from ethyl-2-chloro-2,2-difluoroacetate, serves as a difluoromethylene building block precursor, underlining its role in organic synthesis (Clavel et al., 2000).
Difluoroalkylation and Photocatalysis
- Visible-Light-Driven Difluoroacetylation: Ethyl 2-bromo-2,2-difluoroacetate reacts with alkenes under blue light-emitting diode irradiation, demonstrating its potential in photocatalysis and difluoroalkylation (Furukawa et al., 2020).
- Aromatic Difluoroalkylation: Ethyl 2-bromo-2,2-difluoroacetate is used in the synthesis of difluoroalkylated aromatics, a vital component in pharmaceutical and agrochemical applications. This method showcases its utility in creating structurally diverse compounds (Jung et al., 2014).
Organic Synthesis and Chemical Transformations
- N-Formylation of Amines: Ethyl bromodifluoroacetate acts as an N-formylating agent in the copper-catalyzed N-formylation of amines, demonstrating its versatility in organic transformations (Li et al., 2018).
- Formation of Difluoromethylated Molecules: Difluoroacetaldehyde ethyl hemiacetal, prepared from ethyl difluoroacetate, is a promising reagent for the synthesis of a wide variety of difluoromethylated compounds, underlining its significance in the field of organic chemistry (Kaneko et al., 1993).
Environmental Implications
- Photooxidation of Fluoroacetates: The study of the Cl-initiated photooxidation of fluoroacetates, including this compound, reveals its role in the formation of fluorocarboxylic acids in the atmosphere, highlighting environmental implications (Blanco et al., 2010).
Safety and Hazards
Future Directions
Mechanism of Action
Target of Action
Ethyl difluoroacetate is a chemical compound that primarily targets the respiratory system . It is used in pharmaceutical intermediates, acrylization oxidation, and halogenating reaction of catalyst .
Mode of Action
It is known to interact with its targets in the respiratory system, leading to various changes at the molecular level .
Biochemical Pathways
This compound is involved in several biochemical pathways. It is degraded to difluoroacetic acid by strains of Actinobacteria . This suggests that it may play a role in certain metabolic pathways involving these bacteria.
Pharmacokinetics
Given its use in pharmaceutical intermediates, it is likely that these properties have been optimized to ensure adequate bioavailability .
Result of Action
It is known to have an impact on the respiratory system
Action Environment
The action, efficacy, and stability of this compound can be influenced by various environmental factors. For instance, its degradation to difluoroacetic acid by Actinobacteria suggests that the presence and activity of these bacteria could influence its action . Other factors such as temperature, pH, and the presence of other chemicals could also potentially influence its action.
Biochemical Analysis
Biochemical Properties
Ethyl difluoroacetate plays a role in biochemical reactions, particularly in the acrylization oxidation and halogenating reaction of catalysts
Cellular Effects
It has been noted that when this compound was introduced in a battery system, it led to the decomposition of the electrolyte, affecting the cycle performance of the battery
Molecular Mechanism
It is known to degrade to difluoroacetic acid by strains of Actinobacteria
Temporal Effects in Laboratory Settings
It is known that this compound has a boiling point of 99.2 °C and a density of 1.18 g/mL at 25 °C .
Properties
IUPAC Name |
ethyl 2,2-difluoroacetate | |
---|---|---|
Source | PubChem | |
URL | https://pubchem.ncbi.nlm.nih.gov | |
Description | Data deposited in or computed by PubChem | |
InChI |
InChI=1S/C4H6F2O2/c1-2-8-4(7)3(5)6/h3H,2H2,1H3 | |
Source | PubChem | |
URL | https://pubchem.ncbi.nlm.nih.gov | |
Description | Data deposited in or computed by PubChem | |
InChI Key |
GZKHDVAKKLTJPO-UHFFFAOYSA-N | |
Source | PubChem | |
URL | https://pubchem.ncbi.nlm.nih.gov | |
Description | Data deposited in or computed by PubChem | |
Canonical SMILES |
CCOC(=O)C(F)F | |
Source | PubChem | |
URL | https://pubchem.ncbi.nlm.nih.gov | |
Description | Data deposited in or computed by PubChem | |
Molecular Formula |
C4H6F2O2 | |
Source | PubChem | |
URL | https://pubchem.ncbi.nlm.nih.gov | |
Description | Data deposited in or computed by PubChem | |
DSSTOX Substance ID |
DTXSID0060010 | |
Record name | Ethyl difluoroacetate | |
Source | EPA DSSTox | |
URL | https://comptox.epa.gov/dashboard/DTXSID0060010 | |
Description | DSSTox provides a high quality public chemistry resource for supporting improved predictive toxicology. | |
Molecular Weight |
124.09 g/mol | |
Source | PubChem | |
URL | https://pubchem.ncbi.nlm.nih.gov | |
Description | Data deposited in or computed by PubChem | |
CAS No. |
454-31-9 | |
Record name | Ethyl difluoroacetate | |
Source | CAS Common Chemistry | |
URL | https://commonchemistry.cas.org/detail?cas_rn=454-31-9 | |
Description | CAS Common Chemistry is an open community resource for accessing chemical information. Nearly 500,000 chemical substances from CAS REGISTRY cover areas of community interest, including common and frequently regulated chemicals, and those relevant to high school and undergraduate chemistry classes. This chemical information, curated by our expert scientists, is provided in alignment with our mission as a division of the American Chemical Society. | |
Explanation | The data from CAS Common Chemistry is provided under a CC-BY-NC 4.0 license, unless otherwise stated. | |
Record name | Acetic acid, 2,2-difluoro-, ethyl ester | |
Source | ChemIDplus | |
URL | https://pubchem.ncbi.nlm.nih.gov/substance/?source=chemidplus&sourceid=0000454319 | |
Description | ChemIDplus is a free, web search system that provides access to the structure and nomenclature authority files used for the identification of chemical substances cited in National Library of Medicine (NLM) databases, including the TOXNET system. | |
Record name | Acetic acid, 2,2-difluoro-, ethyl ester | |
Source | EPA Chemicals under the TSCA | |
URL | https://www.epa.gov/chemicals-under-tsca | |
Description | EPA Chemicals under the Toxic Substances Control Act (TSCA) collection contains information on chemicals and their regulations under TSCA, including non-confidential content from the TSCA Chemical Substance Inventory and Chemical Data Reporting. | |
Record name | Ethyl difluoroacetate | |
Source | EPA DSSTox | |
URL | https://comptox.epa.gov/dashboard/DTXSID0060010 | |
Description | DSSTox provides a high quality public chemistry resource for supporting improved predictive toxicology. | |
Record name | Ethyl difluoroacetate | |
Source | European Chemicals Agency (ECHA) | |
URL | https://echa.europa.eu/substance-information/-/substanceinfo/100.006.568 | |
Description | The European Chemicals Agency (ECHA) is an agency of the European Union which is the driving force among regulatory authorities in implementing the EU's groundbreaking chemicals legislation for the benefit of human health and the environment as well as for innovation and competitiveness. | |
Explanation | Use of the information, documents and data from the ECHA website is subject to the terms and conditions of this Legal Notice, and subject to other binding limitations provided for under applicable law, the information, documents and data made available on the ECHA website may be reproduced, distributed and/or used, totally or in part, for non-commercial purposes provided that ECHA is acknowledged as the source: "Source: European Chemicals Agency, http://echa.europa.eu/". Such acknowledgement must be included in each copy of the material. ECHA permits and encourages organisations and individuals to create links to the ECHA website under the following cumulative conditions: Links can only be made to webpages that provide a link to the Legal Notice page. | |
Record name | Ethyl difluoroacetate | |
Source | FDA Global Substance Registration System (GSRS) | |
URL | https://gsrs.ncats.nih.gov/ginas/app/beta/substances/A3R5VZ4KWN | |
Description | The FDA Global Substance Registration System (GSRS) enables the efficient and accurate exchange of information on what substances are in regulated products. Instead of relying on names, which vary across regulatory domains, countries, and regions, the GSRS knowledge base makes it possible for substances to be defined by standardized, scientific descriptions. | |
Explanation | Unless otherwise noted, the contents of the FDA website (www.fda.gov), both text and graphics, are not copyrighted. They are in the public domain and may be republished, reprinted and otherwise used freely by anyone without the need to obtain permission from FDA. Credit to the U.S. Food and Drug Administration as the source is appreciated but not required. | |
Retrosynthesis Analysis
AI-Powered Synthesis Planning: Our tool employs the Template_relevance Pistachio, Template_relevance Bkms_metabolic, Template_relevance Pistachio_ringbreaker, Template_relevance Reaxys, Template_relevance Reaxys_biocatalysis model, leveraging a vast database of chemical reactions to predict feasible synthetic routes.
One-Step Synthesis Focus: Specifically designed for one-step synthesis, it provides concise and direct routes for your target compounds, streamlining the synthesis process.
Accurate Predictions: Utilizing the extensive PISTACHIO, BKMS_METABOLIC, PISTACHIO_RINGBREAKER, REAXYS, REAXYS_BIOCATALYSIS database, our tool offers high-accuracy predictions, reflecting the latest in chemical research and data.
Strategy Settings
Precursor scoring | Relevance Heuristic |
---|---|
Min. plausibility | 0.01 |
Model | Template_relevance |
Template Set | Pistachio/Bkms_metabolic/Pistachio_ringbreaker/Reaxys/Reaxys_biocatalysis |
Top-N result to add to graph | 6 |
Feasible Synthetic Routes
Disclaimer and Information on In-Vitro Research Products
Please be aware that all articles and product information presented on BenchChem are intended solely for informational purposes. The products available for purchase on BenchChem are specifically designed for in-vitro studies, which are conducted outside of living organisms. In-vitro studies, derived from the Latin term "in glass," involve experiments performed in controlled laboratory settings using cells or tissues. It is important to note that these products are not categorized as medicines or drugs, and they have not received approval from the FDA for the prevention, treatment, or cure of any medical condition, ailment, or disease. We must emphasize that any form of bodily introduction of these products into humans or animals is strictly prohibited by law. It is essential to adhere to these guidelines to ensure compliance with legal and ethical standards in research and experimentation.