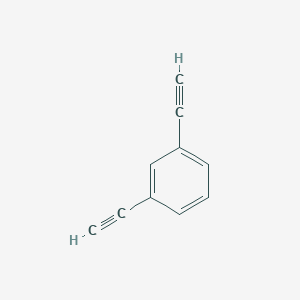
1,3-Diethynylbenzene
Overview
Description
1,3-Diethynylbenzene is an organic compound with the molecular formula C10H6. It consists of a benzene ring substituted with two ethynyl groups at the 1 and 3 positions. This compound is known for its conjugated π-electron system, which imparts unique chemical and physical properties. It is used in various scientific and industrial applications due to its stability and reactivity.
Mechanism of Action
Target of Action
1,3-Diethynylbenzene is a chemical compound with the empirical formula C10H6 . .
Mode of Action
It’s known that this compound can be used to synthesize mono- and di- 1,4-substituted-1,2,3-triazole ligands
Biochemical Pathways
It’s known that the compound can be used to synthesize ligands that have the property to self-assemble into metallo-cyclic structures in the presence of silver ions . .
Result of Action
It’s known that the compound can be used to synthesize ligands that have the property to self-assemble into metallo-cyclic structures
Preparation Methods
1,3-Diethynylbenzene can be synthesized through several methods:
Sonogashira Coupling Reaction: This method involves the coupling of 1,3-dibromobenzene with terminal alkynes in the presence of a palladium catalyst and a copper co-catalyst. The reaction is typically carried out in an inert atmosphere using solvents like tetrahydrofuran or dimethylformamide.
Dehydrohalogenation: Another method involves the dehydrohalogenation of 1,3-dihaloalkenes using strong bases such as potassium tert-butoxide. This reaction results in the formation of this compound.
Industrial Production: Industrially, this compound can be produced through the oxidative coupling of acetylene derivatives in the presence of metal catalysts. This method is advantageous due to its scalability and cost-effectiveness.
Chemical Reactions Analysis
1,3-Diethynylbenzene undergoes various chemical reactions, including:
Oxidation: It can be oxidized to form diketones or carboxylic acids using oxidizing agents like potassium permanganate or chromium trioxide.
Reduction: Reduction of this compound can be achieved using hydrogenation catalysts such as palladium on carbon, leading to the formation of 1,3-dialkylbenzenes.
Substitution: Electrophilic aromatic substitution reactions can occur at the benzene ring, allowing for the introduction of various functional groups. Common reagents include halogens, nitrating agents, and sulfonating agents.
Cycloaddition: The ethynyl groups can participate in cycloaddition reactions, such as the Diels-Alder reaction, to form polycyclic aromatic compounds.
Scientific Research Applications
1,3-Diethynylbenzene has a wide range of applications in scientific research:
Organic Electronics: It is used in the synthesis of conductive polymers and organic semiconductors due to its conjugated π-electron system.
Material Science: The compound is employed in the preparation of carbon nanomaterials and graphitic structures, which have applications in nanotechnology and materials science.
Medicinal Chemistry: It serves as a building block for the synthesis of bioactive molecules and pharmaceutical intermediates.
Photovoltaics: this compound derivatives are used in the development of organic photovoltaic cells for solar energy conversion.
Comparison with Similar Compounds
1,3-Diethynylbenzene can be compared with other similar compounds, such as:
1,4-Diethynylbenzene: This compound has ethynyl groups at the 1 and 4 positions, resulting in different electronic properties and reactivity.
1,2-Diethynylbenzene: With ethynyl groups at the 1 and 2 positions, this compound exhibits unique steric and electronic effects compared to this compound.
1,3,5-Triethynylbenzene: This compound has three ethynyl groups, leading to increased conjugation and potential for forming more complex structures.
This compound is unique due to its specific substitution pattern, which provides a balance between reactivity and stability, making it suitable for a wide range of applications.
Properties
IUPAC Name |
1,3-diethynylbenzene | |
---|---|---|
Source | PubChem | |
URL | https://pubchem.ncbi.nlm.nih.gov | |
Description | Data deposited in or computed by PubChem | |
InChI |
InChI=1S/C10H6/c1-3-9-6-5-7-10(4-2)8-9/h1-2,5-8H | |
Source | PubChem | |
URL | https://pubchem.ncbi.nlm.nih.gov | |
Description | Data deposited in or computed by PubChem | |
InChI Key |
PNXLPYYXCOXPBM-UHFFFAOYSA-N | |
Source | PubChem | |
URL | https://pubchem.ncbi.nlm.nih.gov | |
Description | Data deposited in or computed by PubChem | |
Canonical SMILES |
C#CC1=CC(=CC=C1)C#C | |
Source | PubChem | |
URL | https://pubchem.ncbi.nlm.nih.gov | |
Description | Data deposited in or computed by PubChem | |
Molecular Formula |
C10H6 | |
Source | PubChem | |
URL | https://pubchem.ncbi.nlm.nih.gov | |
Description | Data deposited in or computed by PubChem | |
Related CAS |
25359-90-4 | |
Record name | Benzene, 1,3-diethynyl-, homopolymer | |
Source | CAS Common Chemistry | |
URL | https://commonchemistry.cas.org/detail?cas_rn=25359-90-4 | |
Description | CAS Common Chemistry is an open community resource for accessing chemical information. Nearly 500,000 chemical substances from CAS REGISTRY cover areas of community interest, including common and frequently regulated chemicals, and those relevant to high school and undergraduate chemistry classes. This chemical information, curated by our expert scientists, is provided in alignment with our mission as a division of the American Chemical Society. | |
Explanation | The data from CAS Common Chemistry is provided under a CC-BY-NC 4.0 license, unless otherwise stated. | |
DSSTOX Substance ID |
DTXSID20170491 | |
Record name | Benzene, 1,3-diethynyl- | |
Source | EPA DSSTox | |
URL | https://comptox.epa.gov/dashboard/DTXSID20170491 | |
Description | DSSTox provides a high quality public chemistry resource for supporting improved predictive toxicology. | |
Molecular Weight |
126.15 g/mol | |
Source | PubChem | |
URL | https://pubchem.ncbi.nlm.nih.gov | |
Description | Data deposited in or computed by PubChem | |
CAS No. |
1785-61-1 | |
Record name | Benzene, 1,3-diethynyl- | |
Source | ChemIDplus | |
URL | https://pubchem.ncbi.nlm.nih.gov/substance/?source=chemidplus&sourceid=0001785611 | |
Description | ChemIDplus is a free, web search system that provides access to the structure and nomenclature authority files used for the identification of chemical substances cited in National Library of Medicine (NLM) databases, including the TOXNET system. | |
Record name | Benzene, 1,3-diethynyl- | |
Source | EPA DSSTox | |
URL | https://comptox.epa.gov/dashboard/DTXSID20170491 | |
Description | DSSTox provides a high quality public chemistry resource for supporting improved predictive toxicology. | |
Record name | m-Diethynylbenzene | |
Source | European Chemicals Agency (ECHA) | |
URL | https://echa.europa.eu/information-on-chemicals | |
Description | The European Chemicals Agency (ECHA) is an agency of the European Union which is the driving force among regulatory authorities in implementing the EU's groundbreaking chemicals legislation for the benefit of human health and the environment as well as for innovation and competitiveness. | |
Explanation | Use of the information, documents and data from the ECHA website is subject to the terms and conditions of this Legal Notice, and subject to other binding limitations provided for under applicable law, the information, documents and data made available on the ECHA website may be reproduced, distributed and/or used, totally or in part, for non-commercial purposes provided that ECHA is acknowledged as the source: "Source: European Chemicals Agency, http://echa.europa.eu/". Such acknowledgement must be included in each copy of the material. ECHA permits and encourages organisations and individuals to create links to the ECHA website under the following cumulative conditions: Links can only be made to webpages that provide a link to the Legal Notice page. | |
Retrosynthesis Analysis
AI-Powered Synthesis Planning: Our tool employs the Template_relevance Pistachio, Template_relevance Bkms_metabolic, Template_relevance Pistachio_ringbreaker, Template_relevance Reaxys, Template_relevance Reaxys_biocatalysis model, leveraging a vast database of chemical reactions to predict feasible synthetic routes.
One-Step Synthesis Focus: Specifically designed for one-step synthesis, it provides concise and direct routes for your target compounds, streamlining the synthesis process.
Accurate Predictions: Utilizing the extensive PISTACHIO, BKMS_METABOLIC, PISTACHIO_RINGBREAKER, REAXYS, REAXYS_BIOCATALYSIS database, our tool offers high-accuracy predictions, reflecting the latest in chemical research and data.
Strategy Settings
Precursor scoring | Relevance Heuristic |
---|---|
Min. plausibility | 0.01 |
Model | Template_relevance |
Template Set | Pistachio/Bkms_metabolic/Pistachio_ringbreaker/Reaxys/Reaxys_biocatalysis |
Top-N result to add to graph | 6 |
Feasible Synthetic Routes
Q1: What is the molecular formula, weight, and key spectroscopic data for 1,3-diethynylbenzene?
A1: this compound has the molecular formula C10H6 and a molecular weight of 126.15 g/mol. Spectroscopically, it is characterized by strong IR absorptions due to the C≡C stretching vibrations and characteristic signals in the 1H and 13C NMR spectra.
Q2: What is the significance of this compound's structure in material science?
A2: The rigid, conjugated structure of this compound makes it an attractive building block for constructing polymers and porous materials. [] Its two ethynyl groups can participate in various polymerization reactions, leading to materials with unique properties. [, ]
Q3: How does this compound contribute to the properties of polymers?
A3: When incorporated into polymers, this compound introduces rigidity and extends the π-conjugation system. [] This can enhance properties like thermal stability, mechanical strength, and optical properties. [] For example, silicon-containing polymers with this compound exhibit high thermal stability, with some showing 5% weight loss temperatures exceeding 800°C. []
Q4: What are the advantages of using this compound in creating porous materials?
A4: this compound serves as a building block for microporous hyper-cross-linked polymers. [] These polymers exhibit high surface areas and tuneable pore sizes, making them suitable for applications like gas storage and separation. [, ]
Q5: Does this compound play a role in catalytic processes?
A5: While this compound itself might not be catalytically active, it can be incorporated into structures that are. For instance, it can be used to bridge metal centers in dinuclear complexes, potentially influencing their catalytic activity. []
Q6: How is computational chemistry used in understanding this compound-containing systems?
A6: Computational methods like density functional theory (DFT) help predict and understand the electronic structures, optical properties, and magnetic properties of molecules and materials containing this compound. [, ] These insights are crucial for designing materials with desired properties.
Q7: How do structural modifications to this compound impact the properties of its derivatives?
A7: Introducing substituents onto the benzene ring of this compound can significantly alter the properties of the resulting materials. [, ] For example, adding electron-donating or -withdrawing groups can tune the electronic properties and influence the performance in applications like organic solar cells. []
Q8: What are the potential applications of this compound in different fields?
A8: this compound holds promise for diverse applications, including:
- High-performance materials: Its use in synthesizing heat-resistant polymers and composites is well-documented. [, ]
- Energy storage: Its incorporation into electrode materials for lithium-ion batteries can improve their performance. [, ]
- Gas storage and separation: Porous materials derived from this compound show potential in capturing CO2 and water vapor. []
- Sensors: The sensitivity of this compound derivatives to external stimuli, like metal ions, makes them suitable for sensor development. [, ]
- Biomedical applications: Its use in synthesizing biocompatible polymers makes it relevant for biomedical applications, although this area requires further investigation. []
Q9: What are some future research directions for this compound?
A9: Research on this compound is continuously evolving. Some promising directions include:
- Exploring new polymerization methods to create materials with enhanced properties and functionalities. []
- Developing sustainable and environmentally friendly synthesis and processing techniques. []
- Investigating the potential of this compound derivatives in biomedicine, such as drug delivery and tissue engineering. []
- Utilizing computational modeling to accelerate the discovery and development of new materials with tailored properties. []
Disclaimer and Information on In-Vitro Research Products
Please be aware that all articles and product information presented on BenchChem are intended solely for informational purposes. The products available for purchase on BenchChem are specifically designed for in-vitro studies, which are conducted outside of living organisms. In-vitro studies, derived from the Latin term "in glass," involve experiments performed in controlled laboratory settings using cells or tissues. It is important to note that these products are not categorized as medicines or drugs, and they have not received approval from the FDA for the prevention, treatment, or cure of any medical condition, ailment, or disease. We must emphasize that any form of bodily introduction of these products into humans or animals is strictly prohibited by law. It is essential to adhere to these guidelines to ensure compliance with legal and ethical standards in research and experimentation.