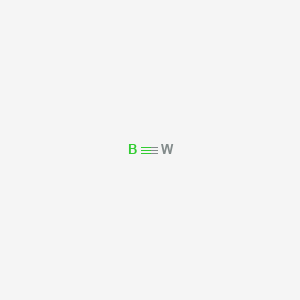
Tungsten boride
Overview
Description
Tungsten boride is a compound formed by the combination of tungsten and boron. It is known for its remarkable hardness, which makes it a material of interest in various industrial applications. Tungsten borides exist in several stoichiometric forms, including tungsten diboride, tungsten tetraboride, and tungsten pentaboride. These compounds exhibit high melting points, excellent mechanical properties, and significant resistance to oxidation and wear .
Mechanism of Action
Target of Action
Tungsten borides are a unique class of transition-metal light-element compounds known for their superior mechanical properties . They are primarily targeted for their hardness, high melting points, and strong resistance to oxidation and acids . The thermodynamic stability of various compositions of tungsten borides has been established, and a large number of metastable phases have been identified .
Mode of Action
The interaction of tungsten boride with its targets primarily involves the formation of complex crystal structures . The formation of these structures is influenced by the versatile ability of boron atoms to form sp-, sp2-, sp3-hybridized and even multicenter bonds . The formation of W2B, for instance, is initiated at approximately 1000° C in excess of tungsten content .
Biochemical Pathways
The biochemical pathways affected by this compound are largely related to its structural formation. The highest boride of tungsten, previously denoted WB4 with a three-dimensional boron network, is identified as WB3 with two-dimensional boron nets . The mechanical properties of tungsten borides correlate with their formation energies .
Pharmacokinetics
Its superior mechanical properties, such as ultra-hardness, high melting points, and strong resistance to oxidation and acids, suggest that it may have significant bioavailability .
Result of Action
The molecular and cellular effects of this compound’s action are primarily observed in its structural formations. For instance, the formation of WB3 with two-dimensional boron nets is a result of this compound’s action . Furthermore, the mechanical properties of tungsten borides, such as their hardness, correlate with their formation energies .
Action Environment
The action of this compound is influenced by environmental factors such as temperature and pressure. For example, the formation of W2B is initiated at approximately 1000° C in excess of tungsten content . Additionally, the presence of boron atoms and their ability to form various types of bonds also influence the action of this compound .
Preparation Methods
Synthetic Routes and Reaction Conditions: Tungsten borides can be synthesized through various methods, including:
Boriding Reaction: This involves reacting tungsten powder with boron nitride at high temperatures (around 1723 K) in an argon atmosphere or vacuum.
Solid-State Reaction: Tungsten borides can be synthesized by reacting tungsten and boron powders under vacuum in a graphite element furnace.
Mechanical Activation and Chemical Homogenization: This method involves grinding tungsten and boron powders in a planetary ball mill, followed by drying at specific temperatures depending on the boron source used.
Industrial Production Methods: Industrial production of tungsten borides typically involves high-temperature solid-state reactions and boriding processes. These methods are scalable and can produce large quantities of tungsten borides with high purity and controlled stoichiometry .
Types of Reactions:
Oxidation: Tungsten borides undergo oxidation at temperatures above 600°C, forming tungsten trioxide and boron oxides.
Reduction: Tungsten borides can be reduced to elemental tungsten and boron under specific conditions.
Substitution: Tungsten borides can participate in substitution reactions where boron atoms are replaced by other elements.
Common Reagents and Conditions:
Oxidation: Oxygen or air at high temperatures.
Reduction: Hydrogen or other reducing agents at elevated temperatures.
Substitution: Various metal halides or other boron-containing compounds.
Major Products Formed:
Oxidation: Tungsten trioxide and boron oxides.
Reduction: Elemental tungsten and boron.
Substitution: New boride compounds with substituted elements.
Scientific Research Applications
Tungsten borides have a wide range of scientific research applications due to their unique properties:
Chemistry: Used as catalysts in hydrogen evolution reactions and other electrocatalytic processes.
Medicine: Investigated for use in medical implants and prosthetics.
Industry: Utilized in cutting tools, abrasives, and coatings due to their high hardness and wear resistance.
Comparison with Similar Compounds
- Molybdenum boride
- Chromium boride
- Titanium boride
- Vanadium boride
- Zirconium boride
Tungsten borides stand out due to their exceptional hardness and stability, making them highly valuable in both scientific research and industrial applications.
Properties
IUPAC Name |
boranylidynetungsten | |
---|---|---|
Source | PubChem | |
URL | https://pubchem.ncbi.nlm.nih.gov | |
Description | Data deposited in or computed by PubChem | |
InChI |
InChI=1S/B.W | |
Source | PubChem | |
URL | https://pubchem.ncbi.nlm.nih.gov | |
Description | Data deposited in or computed by PubChem | |
InChI Key |
JEEHQNXCPARQJS-UHFFFAOYSA-N | |
Source | PubChem | |
URL | https://pubchem.ncbi.nlm.nih.gov | |
Description | Data deposited in or computed by PubChem | |
Canonical SMILES |
B#[W] | |
Source | PubChem | |
URL | https://pubchem.ncbi.nlm.nih.gov | |
Description | Data deposited in or computed by PubChem | |
Molecular Formula |
BW | |
Source | PubChem | |
URL | https://pubchem.ncbi.nlm.nih.gov | |
Description | Data deposited in or computed by PubChem | |
Molecular Weight |
194.65 g/mol | |
Source | PubChem | |
URL | https://pubchem.ncbi.nlm.nih.gov | |
Description | Data deposited in or computed by PubChem | |
Physical Description |
Grey odorless powder; [MSDSonline] Insoluble in water; [Hawley] | |
Record name | Tungsten boride | |
Source | Haz-Map, Information on Hazardous Chemicals and Occupational Diseases | |
URL | https://haz-map.com/Agents/8701 | |
Description | Haz-Map® is an occupational health database designed for health and safety professionals and for consumers seeking information about the adverse effects of workplace exposures to chemical and biological agents. | |
Explanation | Copyright (c) 2022 Haz-Map(R). All rights reserved. Unless otherwise indicated, all materials from Haz-Map are copyrighted by Haz-Map(R). No part of these materials, either text or image may be used for any purpose other than for personal use. Therefore, reproduction, modification, storage in a retrieval system or retransmission, in any form or by any means, electronic, mechanical or otherwise, for reasons other than personal use, is strictly prohibited without prior written permission. | |
CAS No. |
12007-09-9 | |
Record name | Tungsten boride (WB) | |
Source | CAS Common Chemistry | |
URL | https://commonchemistry.cas.org/detail?cas_rn=12007-09-9 | |
Description | CAS Common Chemistry is an open community resource for accessing chemical information. Nearly 500,000 chemical substances from CAS REGISTRY cover areas of community interest, including common and frequently regulated chemicals, and those relevant to high school and undergraduate chemistry classes. This chemical information, curated by our expert scientists, is provided in alignment with our mission as a division of the American Chemical Society. | |
Explanation | The data from CAS Common Chemistry is provided under a CC-BY-NC 4.0 license, unless otherwise stated. | |
Record name | Tungsten boride (WB) | |
Source | ChemIDplus | |
URL | https://pubchem.ncbi.nlm.nih.gov/substance/?source=chemidplus&sourceid=0012007099 | |
Description | ChemIDplus is a free, web search system that provides access to the structure and nomenclature authority files used for the identification of chemical substances cited in National Library of Medicine (NLM) databases, including the TOXNET system. | |
Record name | Tungsten boride (WB) | |
Source | EPA Chemicals under the TSCA | |
URL | https://www.epa.gov/chemicals-under-tsca | |
Description | EPA Chemicals under the Toxic Substances Control Act (TSCA) collection contains information on chemicals and their regulations under TSCA, including non-confidential content from the TSCA Chemical Substance Inventory and Chemical Data Reporting. | |
Record name | Tungsten boride | |
Source | European Chemicals Agency (ECHA) | |
URL | https://echa.europa.eu/substance-information/-/substanceinfo/100.031.349 | |
Description | The European Chemicals Agency (ECHA) is an agency of the European Union which is the driving force among regulatory authorities in implementing the EU's groundbreaking chemicals legislation for the benefit of human health and the environment as well as for innovation and competitiveness. | |
Explanation | Use of the information, documents and data from the ECHA website is subject to the terms and conditions of this Legal Notice, and subject to other binding limitations provided for under applicable law, the information, documents and data made available on the ECHA website may be reproduced, distributed and/or used, totally or in part, for non-commercial purposes provided that ECHA is acknowledged as the source: "Source: European Chemicals Agency, http://echa.europa.eu/". Such acknowledgement must be included in each copy of the material. ECHA permits and encourages organisations and individuals to create links to the ECHA website under the following cumulative conditions: Links can only be made to webpages that provide a link to the Legal Notice page. | |
Q1: What are the notable properties of tungsten borides?
A1: Tungsten borides are a class of materials known for their impressive hardness, high melting points, chemical inertness, and excellent resistance to thermal shock and corrosion. []
Q2: What is the structural characterization of tungsten borides?
A2: Tungsten borides exist in various stoichiometries, each exhibiting unique crystal structures. For instance:
- WB: Can adopt tetragonal or orthorhombic modifications, both exhibiting superconductivity at low temperatures. []
- W2B4: Formerly misidentified as W2B5, crystallizes in a unique structure and exhibits superconductivity. []
- WB2+x, WB3+x: These boron-rich phases have been theoretically predicted and are of great interest for their potential superhardness. []
- WB5-x: This recently synthesized boron-rich phase exhibits excellent mechanical properties and is structurally similar to the predicted WB5. []
Q3: Is there spectroscopic data available for tungsten borides?
A3: While specific spectroscopic data isn't extensively detailed in the provided research, techniques like X-ray diffraction (XRD), neutron diffraction, and X-ray photoelectron spectroscopy (XPS) have been crucial for characterizing the crystal structures, phase compositions, and chemical bonding in these materials. [, , ]
Q4: What are the potential applications of tungsten borides?
A4: Tungsten borides have garnered interest for a variety of applications:
- Radiation Shielding: Their high density and ability to attenuate gamma rays and neutrons make them attractive for radiation protection in nuclear power plants. [, ]
- Cutting Tools: Their exceptional hardness makes them suitable for wear-resistant coatings on cutting tools and drill bits. [, ]
- Lithium-Ion Batteries: Tungsten borides like WB2 and WB4 show promise as anode materials due to their electrical conductivity and chemical stability. []
- High-Temperature Applications: Their high melting points and thermal shock resistance make them suitable for applications involving extreme temperatures. []
- Solar Energy: WB-based thin films have shown potential for use as thermally stable broadband absorbers in solar energy applications. [, ]
Q5: How does the performance of tungsten boride compare to other materials in these applications?
A5:
- Radiation Shielding: Tungsten borides, particularly W2B5, outperform monolithic tungsten and offer comparable or even superior shielding performance to water-cooled shields. []
- Cutting Tools: WB coatings significantly improve the drilling rate of diamond/Fe-Ni composite drill bits compared to uncoated diamond bits. []
- Lithium-Ion Batteries: Nanostructured this compound electrodes show improved lithium-ion reaction properties compared to bulk materials, enhancing cyclability and high-rate performance. []
Q6: How do the properties of different this compound compositions affect their performance?
A6:
- Boron Content and Hardness: Increasing boron content generally leads to higher hardness in tungsten borides. For instance, WB5-x is significantly harder than the WB2 and WB3 phases. []
- Structure and Mechanical Properties: The unique bonding configurations and deformation modes within different tungsten borides, influenced by boron concentration, result in diverse stress responses and indentation strengths. []
Q7: How are tungsten borides synthesized?
A7: Several methods are employed for synthesizing tungsten borides:
- Self-propagating High-temperature Synthesis (SHS): This method utilizes the exothermic reaction between tungsten oxide (WO3), boron oxide (B2O3), and magnesium (Mg) to produce tungsten borides. [, ]
- High-Pressure Synthesis: This technique utilizes high pressures to stabilize the formation of boron-rich tungsten borides, overcoming their thermodynamic unfavorability at ambient pressure. []
- Electrochemical Synthesis: this compound phases can be synthesized electrochemically using molten salt systems containing boron oxide and tungsten oxide as reactants. [, ]
- Mechanical Milling: High-energy ball milling can induce the formation of tungsten borides from elemental tungsten and boron powders at room temperature. [, ]
Q8: What factors influence the synthesis of specific this compound phases?
A8:
- Reactant Ratios: The stoichiometry of the final product is heavily influenced by the initial molar ratios of the reactants. [, ]
- Temperature and Pressure: The synthesis of specific phases often requires specific temperature and pressure conditions. For example, WB4 is predicted to be stable only above 1 GPa. []
- Atmosphere: The atmosphere during heat treatment can impact the formation and homogeneity of this compound phases. []
Q9: How stable are tungsten borides under different conditions?
A9:
- Thermal Stability: Tungsten borides exhibit excellent thermal stability, with some compositions remaining stable even at very high temperatures. []
- Chemical Stability: They are highly resistant to corrosion and chemical attack, making them suitable for harsh environments. [, ]
- Radiation Stability: While tungsten borides demonstrate good radiation resistance, prolonged exposure can lead to transmutation and the production of helium gas, potentially impacting their structural integrity. []
Q10: How is computational chemistry used in this compound research?
A10: Computational methods, particularly density functional theory (DFT) calculations, are invaluable for:
- Predicting Crystal Structures: DFT calculations are used to predict the crystal structures of novel tungsten borides, such as WB5, and to refine the structures of known phases. [, , ]
- Calculating Mechanical Properties: DFT can predict the mechanical properties of tungsten borides, including their hardness, elastic constants, and fracture toughness, aiding in the identification of potentially superhard materials. [, , ]
- Understanding Stability: Computational methods help determine the thermodynamic stability of different this compound phases under various conditions. [, ]
- Investigating Defect Properties: DFT calculations are used to study the energetics of intrinsic point defects and hydrogen behavior in tungsten borides, providing insights into their irradiation resistance. []
Q11: Have any QSAR models been developed for tungsten borides?
A11: The provided research doesn't mention the development of Quantitative Structure-Activity Relationship (QSAR) models for tungsten borides. QSAR models are typically used for predicting the biological activity of molecules and are less relevant to the properties of inorganic materials like tungsten borides.
Q12: What are the key findings regarding the interaction of tungsten borides with other materials?
A12:
- Composite Formation: Tungsten borides can be successfully incorporated into composite materials, enhancing their mechanical and wear properties. For example, adding this compound to silicon nitride improves wear resistance and reduces friction. []
- Coating Applications: this compound coatings on diamond particles improve adhesion to metal matrices in composites, enhancing bending strength and cutting performance. []
- Interfacial Interactions: Understanding the interfacial interactions between this compound coatings and substrates is crucial for optimizing their adhesion and performance in various applications. [, ]
Q13: What are the future directions for this compound research?
A13: Promising research avenues include:
- Synthesizing Novel Phases: Exploring new synthesis techniques to achieve the elusive WB5 phase and other theoretically predicted boron-rich tungsten borides. []
- Optimizing Properties: Tailoring the composition and microstructure of tungsten borides to optimize their properties for specific applications, such as radiation shielding or high-temperature environments. [, ]
- Developing Scalable Production: Developing cost-effective and scalable methods for producing high-quality this compound powders and coatings. []
- Exploring New Applications: Investigating the potential of tungsten borides in emerging fields such as energy storage, catalysis, and aerospace engineering. [, ]
Disclaimer and Information on In-Vitro Research Products
Please be aware that all articles and product information presented on BenchChem are intended solely for informational purposes. The products available for purchase on BenchChem are specifically designed for in-vitro studies, which are conducted outside of living organisms. In-vitro studies, derived from the Latin term "in glass," involve experiments performed in controlled laboratory settings using cells or tissues. It is important to note that these products are not categorized as medicines or drugs, and they have not received approval from the FDA for the prevention, treatment, or cure of any medical condition, ailment, or disease. We must emphasize that any form of bodily introduction of these products into humans or animals is strictly prohibited by law. It is essential to adhere to these guidelines to ensure compliance with legal and ethical standards in research and experimentation.