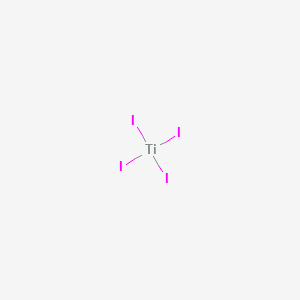
Titanium(IV) iodide
- Click on QUICK INQUIRY to receive a quote from our team of experts.
- With the quality product at a COMPETITIVE price, you can focus more on your research.
Overview
Description
Mechanism of Action
Target of Action
Titanium(IV) iodide, also known as Titanium tetraiodide, is a rare molecular binary metal iodide, consisting of isolated molecules of tetrahedral Titanium(IV) centers It’s known that this compound is used in the van arkel–de boer process for the purification of titanium .
Biochemical Pathways
This compound forms adducts with lewis bases, and it can also be reduced . When the reduction is conducted in the presence of Titanium metal, one obtains polymeric Titanium(III) and Titanium(II) derivatives .
Pharmacokinetics
It’s known that this compound is a volatile solid , suggesting that it could potentially be absorbed and distributed in the body through inhalation. More research is needed to fully understand the pharmacokinetics of this compound.
Result of Action
It’s known that this compound can form adducts with lewis bases and can be reduced . These reactions could potentially have effects at the molecular and cellular level.
Action Environment
Environmental factors can influence the action, efficacy, and stability of this compound. For instance, this compound can be distilled without decomposition at one atmosphere , suggesting that atmospheric pressure can influence its stability. Furthermore, this compound is known to undergo violent hydrolysis , indicating that its interaction with water can significantly affect its stability and reactivity.
Biochemical Analysis
Biochemical Properties
Titanium tetraiodide plays a significant role in various biochemical reactions due to its ability to form adducts with Lewis bases and its mild Lewis acidity . It interacts with enzymes, proteins, and other biomolecules primarily through iodotitanation, reduction, and Lewis acid catalysis . For instance, titanium tetraiodide can induce catalytic aldol and Mannich-type reactions, which are essential in organic synthesis . The compound’s ability to form adducts with Lewis bases allows it to interact with various biomolecules, facilitating biochemical transformations.
Cellular Effects
Titanium tetraiodide influences cell function by affecting cell signaling pathways, gene expression, and cellular metabolism. Its mild Lewis acidity enables it to participate in catalytic reactions that can alter cellular processes . For example, titanium tetraiodide can promote the formation of halogenated cyclopent-2-enone derivatives, which can impact cellular metabolism . Additionally, its ability to form adducts with Lewis bases allows it to interact with cellular proteins and enzymes, potentially affecting gene expression and cell signaling pathways.
Molecular Mechanism
At the molecular level, titanium tetraiodide exerts its effects through binding interactions with biomolecules, enzyme inhibition or activation, and changes in gene expression . The compound’s ability to form adducts with Lewis bases allows it to interact with enzymes and proteins, leading to enzyme inhibition or activation . For instance, titanium tetraiodide can induce the catalytic aldol and Mannich-type reactions by acting as a Lewis acid . These interactions can result in changes in gene expression and cellular function.
Temporal Effects in Laboratory Settings
In laboratory settings, the effects of titanium tetraiodide can change over time due to its stability and degradation properties . The compound is known to be stable under standard conditions but can undergo hydrolysis in the presence of water . Long-term exposure to titanium tetraiodide in in vitro or in vivo studies has shown that it can cause changes in cellular function, including alterations in gene expression and cellular metabolism . The stability and degradation of titanium tetraiodide are crucial factors in determining its long-term effects on cellular function.
Dosage Effects in Animal Models
The effects of titanium tetraiodide vary with different dosages in animal models. At low doses, the compound can promote beneficial biochemical reactions, such as catalytic aldol and Mannich-type reactions . At high doses, titanium tetraiodide can exhibit toxic or adverse effects, including enzyme inhibition and disruption of cellular processes . Studies have shown that there are threshold effects for titanium tetraiodide, where the compound’s beneficial effects are observed at lower doses, while toxic effects are seen at higher doses .
Metabolic Pathways
Titanium tetraiodide is involved in various metabolic pathways, interacting with enzymes and cofactors to facilitate biochemical reactions . The compound’s ability to form adducts with Lewis bases allows it to participate in catalytic reactions, such as aldol and Mannich-type reactions . These interactions can affect metabolic flux and metabolite levels, leading to changes in cellular metabolism . Titanium tetraiodide’s role in metabolic pathways is crucial for understanding its biochemical properties and effects on cellular function.
Transport and Distribution
Within cells and tissues, titanium tetraiodide is transported and distributed through interactions with transporters and binding proteins . The compound’s ability to form adducts with Lewis bases allows it to bind to specific proteins, facilitating its transport and distribution . Titanium tetraiodide’s localization and accumulation within cells can impact its biochemical effects, influencing cellular processes and function .
Subcellular Localization
Titanium tetraiodide’s subcellular localization is determined by its interactions with targeting signals and post-translational modifications . The compound can be directed to specific compartments or organelles within the cell, where it exerts its biochemical effects . For instance, titanium tetraiodide can localize to the cytoplasm or nucleus, affecting gene expression and cellular metabolism . Understanding the subcellular localization of titanium tetraiodide is essential for elucidating its mechanism of action and effects on cellular function.
Preparation Methods
Titanium(IV) iodide can be synthesized through several methods:
-
Direct Combination of Elements: : Titanium metal reacts with iodine at elevated temperatures (around 425°C) to form this compound: [ \text{Ti} + 2 \text{I}_2 \rightarrow \text{TiI}_4 ] This reaction is typically conducted in a tube furnace .
-
Exchange Reaction: : Titanium tetrachloride reacts with hydrogen iodide to produce this compound and hydrogen chloride: [ \text{TiCl}_4 + 4 \text{HI} \rightarrow \text{TiI}_4 + 4 \text{HCl} ] This method is useful for producing this compound in a controlled laboratory setting .
-
Oxide-Iodide Exchange: : Titanium dioxide reacts with aluminum iodide to form this compound and aluminum oxide: [ 3 \text{TiO}_2 + 4 \text{AlI}_3 \rightarrow 3 \text{TiI}_4 + 2 \text{Al}_2\text{O}_3 ] This method is often used in industrial settings .
Chemical Reactions Analysis
Titanium(IV) iodide undergoes various chemical reactions, including:
-
Hydrolysis: : this compound reacts violently with water, leading to hydrolysis and the formation of titanium dioxide and hydrogen iodide: [ \text{TiI}_4 + 2 \text{H}_2\text{O} \rightarrow \text{TiO}_2 + 4 \text{HI} ]
-
Reduction: : this compound can be reduced to form lower oxidation state titanium compounds. For example, in the presence of titanium metal, it can form polymeric titanium(III) and titanium(II) derivatives .
-
Formation of Adducts: : this compound forms adducts with Lewis bases, which can be used in various chemical syntheses .
Scientific Research Applications
Titanium(IV) iodide has several applications in scientific research:
-
Chemical Vapor Deposition (CVD): : It is used in the CVD process to produce titanium nitride films, which have optimized characteristics for various industrial applications .
-
Nanoparticle Synthesis: : this compound is used in the synthesis of titanium dioxide nanoparticles, which are important in catalysis and materials design .
-
Material Science: : The compound is used in the production of high-purity titanium films through the van Arkel–de Boer process .
Comparison with Similar Compounds
Titanium(IV) iodide can be compared with other titanium halides, such as:
-
Titanium(IV) Chloride (TiCl₄): : Unlike this compound, titanium(IV) chloride is a colorless liquid at room temperature and has a lower melting point (-24°C compared to 150°C for TiI₄) .
-
Titanium(IV) Bromide (TiBr₄): : This compound is similar to this compound but has different physical properties, such as a lower melting point and different solubility characteristics .
-
Titanium(IV) Fluoride (TiF₄): : Titanium(IV) fluoride is a white solid with different reactivity and applications compared to this compound .
This compound is unique due to its high molecular weight and specific applications in the purification of titanium and the synthesis of specialized materials.
Properties
CAS No. |
7720-83-4 |
---|---|
Molecular Formula |
I4Ti |
Molecular Weight |
555.485 g/mol |
IUPAC Name |
titanium(4+);tetraiodide |
InChI |
InChI=1S/4HI.Ti/h4*1H;/q;;;;+4/p-4 |
InChI Key |
NLLZTRMHNHVXJJ-UHFFFAOYSA-J |
SMILES |
[Ti](I)(I)(I)I |
Canonical SMILES |
[Ti+4].[I-].[I-].[I-].[I-] |
7720-83-4 | |
Origin of Product |
United States |
Disclaimer and Information on In-Vitro Research Products
Please be aware that all articles and product information presented on BenchChem are intended solely for informational purposes. The products available for purchase on BenchChem are specifically designed for in-vitro studies, which are conducted outside of living organisms. In-vitro studies, derived from the Latin term "in glass," involve experiments performed in controlled laboratory settings using cells or tissues. It is important to note that these products are not categorized as medicines or drugs, and they have not received approval from the FDA for the prevention, treatment, or cure of any medical condition, ailment, or disease. We must emphasize that any form of bodily introduction of these products into humans or animals is strictly prohibited by law. It is essential to adhere to these guidelines to ensure compliance with legal and ethical standards in research and experimentation.