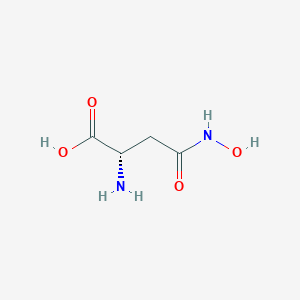
L-Aspartic acid beta-hydroxamate
Overview
Description
L-Aspartic acid beta-hydroxamate is a derivative of the amino acid L-asparagine, characterized by the presence of a hydroxyl group attached to the nitrogen atom of the asparagine molecule
Scientific Research Applications
L-Aspartic acid beta-hydroxamate has diverse applications in scientific research:
Chemistry: Used as a precursor in the synthesis of complex organic molecules.
Biology: Studied for its role in metabolic pathways and enzyme interactions.
Medicine: Investigated for potential therapeutic applications, including cancer treatment.
Industry: Utilized in the production of pharmaceuticals and as a catalyst in chemical reactions.
Mechanism of Action
Safety and Hazards
Future Directions
Research is ongoing to identify and characterize potential new L-asparaginase therapeutics, from existing or novel prokaryotic and eukaryotic sources, including mutations to improve function . Future trends in L-asparaginase production, including the integration of CRISPR and machine learning tools are being discussed .
Biochemical Analysis
Biochemical Properties
L-Aspartic acid beta-hydroxamate interacts with several enzymes and proteins. It is known to inhibit serine racemase, an enzyme involved in the conversion of L-serine to D-serine . The nature of this interaction is inhibitory, meaning that the presence of this compound reduces the activity of serine racemase .
Cellular Effects
The effects of this compound on cells are primarily related to its role as a serine racemase inhibitor. By inhibiting this enzyme, this compound can influence cell function by altering the levels of D-serine, a key modulator of neurotransmission and a co-agonist of the NMDA receptor
Molecular Mechanism
The molecular mechanism of action of this compound involves its binding to the active site of the serine racemase enzyme, thereby inhibiting its activity This results in a decrease in the conversion of L-serine to D-serine
Metabolic Pathways
This compound is involved in the metabolic pathway related to the conversion of L-serine to D-serine, where it acts as an inhibitor of the enzyme serine racemase
Preparation Methods
Synthetic Routes and Reaction Conditions: L-Aspartic acid beta-hydroxamate can be synthesized through several methods. One common approach involves the hydroxylation of L-asparagine using specific reagents under controlled conditions. The reaction typically requires a catalyst and is conducted at a specific temperature to ensure the selective addition of the hydroxyl group to the nitrogen atom.
Industrial Production Methods: In an industrial setting, the production of this compound may involve biocatalysis using asparagine synthetase enzymes. This method is advantageous due to its high efficiency and environmentally friendly nature. The reaction conditions, such as pH, temperature, and substrate concentration, are optimized to maximize yield and purity.
Chemical Reactions Analysis
Types of Reactions: L-Aspartic acid beta-hydroxamate undergoes various chemical reactions, including:
Oxidation: The hydroxyl group can be oxidized to form corresponding oxo derivatives.
Reduction: The compound can be reduced to form L-asparagine.
Substitution: The hydroxyl group can be substituted with other functional groups under specific conditions.
Common Reagents and Conditions:
Oxidation: Common oxidizing agents include hydrogen peroxide and potassium permanganate.
Reduction: Reducing agents such as sodium borohydride are used.
Substitution: Reagents like acyl chlorides or alkyl halides can be employed for substitution reactions.
Major Products:
Oxidation: Formation of oxo derivatives.
Reduction: Conversion back to L-asparagine.
Substitution: Formation of various substituted derivatives depending on the reagent used.
Comparison with Similar Compounds
L-Asparagine: The parent compound, lacking the hydroxyl group.
N-Hydroxy-L-glutamine: Another hydroxylated amino acid derivative.
N-Hydroxy-L-lysine: A hydroxylated derivative of lysine.
Uniqueness: L-Aspartic acid beta-hydroxamate is unique due to its specific hydroxylation pattern, which imparts distinct chemical and biological properties. Its ability to undergo selective reactions and its potential therapeutic applications set it apart from other similar compounds.
Properties
IUPAC Name |
(2S)-2-amino-4-(hydroxyamino)-4-oxobutanoic acid | |
---|---|---|
Source | PubChem | |
URL | https://pubchem.ncbi.nlm.nih.gov | |
Description | Data deposited in or computed by PubChem | |
InChI |
InChI=1S/C4H8N2O4/c5-2(4(8)9)1-3(7)6-10/h2,10H,1,5H2,(H,6,7)(H,8,9)/t2-/m0/s1 | |
Source | PubChem | |
URL | https://pubchem.ncbi.nlm.nih.gov | |
Description | Data deposited in or computed by PubChem | |
InChI Key |
ZBYVTTSIVDYQSO-REOHCLBHSA-N | |
Source | PubChem | |
URL | https://pubchem.ncbi.nlm.nih.gov | |
Description | Data deposited in or computed by PubChem | |
Canonical SMILES |
C(C(C(=O)O)N)C(=O)NO | |
Source | PubChem | |
URL | https://pubchem.ncbi.nlm.nih.gov | |
Description | Data deposited in or computed by PubChem | |
Isomeric SMILES |
C([C@@H](C(=O)O)N)C(=O)NO | |
Source | PubChem | |
URL | https://pubchem.ncbi.nlm.nih.gov | |
Description | Data deposited in or computed by PubChem | |
Molecular Formula |
C4H8N2O4 | |
Source | PubChem | |
URL | https://pubchem.ncbi.nlm.nih.gov | |
Description | Data deposited in or computed by PubChem | |
Molecular Weight |
148.12 g/mol | |
Source | PubChem | |
URL | https://pubchem.ncbi.nlm.nih.gov | |
Description | Data deposited in or computed by PubChem | |
CAS No. |
1955-68-6, 8029-76-3 | |
Record name | L-Asparagine, N-hydroxy- | |
Source | ChemIDplus | |
URL | https://pubchem.ncbi.nlm.nih.gov/substance/?source=chemidplus&sourceid=0001955686 | |
Description | ChemIDplus is a free, web search system that provides access to the structure and nomenclature authority files used for the identification of chemical substances cited in National Library of Medicine (NLM) databases, including the TOXNET system. | |
Record name | Hydroxylated lecithin | |
Source | Human Metabolome Database (HMDB) | |
URL | http://www.hmdb.ca/metabolites/HMDB0032332 | |
Description | The Human Metabolome Database (HMDB) is a freely available electronic database containing detailed information about small molecule metabolites found in the human body. | |
Explanation | HMDB is offered to the public as a freely available resource. Use and re-distribution of the data, in whole or in part, for commercial purposes requires explicit permission of the authors and explicit acknowledgment of the source material (HMDB) and the original publication (see the HMDB citing page). We ask that users who download significant portions of the database cite the HMDB paper in any resulting publications. | |
Retrosynthesis Analysis
AI-Powered Synthesis Planning: Our tool employs the Template_relevance Pistachio, Template_relevance Bkms_metabolic, Template_relevance Pistachio_ringbreaker, Template_relevance Reaxys, Template_relevance Reaxys_biocatalysis model, leveraging a vast database of chemical reactions to predict feasible synthetic routes.
One-Step Synthesis Focus: Specifically designed for one-step synthesis, it provides concise and direct routes for your target compounds, streamlining the synthesis process.
Accurate Predictions: Utilizing the extensive PISTACHIO, BKMS_METABOLIC, PISTACHIO_RINGBREAKER, REAXYS, REAXYS_BIOCATALYSIS database, our tool offers high-accuracy predictions, reflecting the latest in chemical research and data.
Strategy Settings
Precursor scoring | Relevance Heuristic |
---|---|
Min. plausibility | 0.01 |
Model | Template_relevance |
Template Set | Pistachio/Bkms_metabolic/Pistachio_ringbreaker/Reaxys/Reaxys_biocatalysis |
Top-N result to add to graph | 6 |
Feasible Synthetic Routes
Q1: What is the primary mechanism of action of L-Aspartic acid beta-hydroxamate?
A1: this compound acts as an inhibitor for various enzymes. Notably, it demonstrates competitive inhibition against angiotensin-converting enzyme (ACE) [] and serine racemase (SR) []. In the case of SR, it directly interacts with the pyridoxal-5'-phosphate (PLP) cofactor, hindering the enzyme's activity []. It also inhibits assimilatory nitrate reductase (ANR) activity in soil, likely due to its resemblance to glutamine, which is thought to be the actual inhibitor after being formed by microbial assimilation of ammonium [].
Q2: Are there any amino acid residues crucial for the interaction of this compound with its target enzymes?
A3: While not directly related to this compound binding, studies on Escherichia coli asparaginase II (EcA2), an enzyme that utilizes this compound as a substrate, provide insights into potential interactions. Research suggests that the hydroxyl group of Threonine 12 (Thr12) in EcA2 is directly involved in the catalytic mechanism, potentially interacting with a transition state or intermediate formed during the breakdown of this compound [].
Q3: Does modifying the structure of this compound affect its activity?
A4: Yes, structural modifications significantly impact the activity of this compound. For example, replacing the hydroxamate group with a methyl ester on L-Glutamic acid completely abolishes its inhibitory effect on ANR activity, suggesting that the free-acid form of the hydroxamate is essential for inhibition []. Additionally, some aliphatic hydroxamic acids, structurally similar to this compound, exhibit non-specific inhibition of PLP-dependent enzymes due to irreversible aldoxime formation with the PLP cofactor [].
Disclaimer and Information on In-Vitro Research Products
Please be aware that all articles and product information presented on BenchChem are intended solely for informational purposes. The products available for purchase on BenchChem are specifically designed for in-vitro studies, which are conducted outside of living organisms. In-vitro studies, derived from the Latin term "in glass," involve experiments performed in controlled laboratory settings using cells or tissues. It is important to note that these products are not categorized as medicines or drugs, and they have not received approval from the FDA for the prevention, treatment, or cure of any medical condition, ailment, or disease. We must emphasize that any form of bodily introduction of these products into humans or animals is strictly prohibited by law. It is essential to adhere to these guidelines to ensure compliance with legal and ethical standards in research and experimentation.