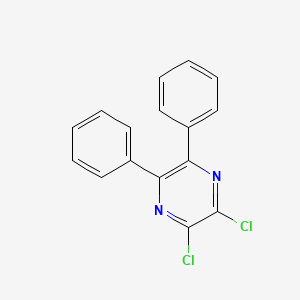
2,3-Dichloro-5,6-diphenylpyrazine
Overview
Description
Preparation Methods
Chlorination of 5,6-Diphenylpyrazine Precursors
The primary synthetic route to 2,3-Dichloro-5,6-diphenylpyrazine involves chlorination of the corresponding 5,6-diphenylpyrazine or its hydroxylated derivatives using chlorinating agents such as phosphoryl chloride or trichlorophosphate.
- Reaction Conditions:
- Use of trichlorophosphate as chlorinating agent
- Heating the reaction mixture for approximately 1 hour
- Typical yields reported are around 86% under optimized conditions
- Reference: Watahiki et al. (1982) demonstrated this method with high efficiency in the Journal of Heterocyclic Chemistry.
This method is notable for its straightforward approach and relatively high yield, making it suitable for laboratory-scale synthesis.
Chlorination via Hydroxypyrazine Intermediates
An alternative and industrially relevant method involves the chlorination of 1-hydroxy-2(1H)-pyrazinones or related hydroxypyrazine derivatives using phosphoryl chloride:
- Process Highlights:
- Conversion of hydroxypyrazine intermediates to dichloropyrazine derivatives through chlorination
- Avoids hazardous nitration steps common in other synthetic routes, reducing explosion risk and lowering production costs
- Reaction temperatures are controlled, often cooled to below 5°C to isolate solid intermediates
- The process yields high purity products with yields up to 92% for related intermediates
- Analytical Techniques:
- Purity and structure confirmed by high-performance liquid chromatography (HPLC) and proton nuclear magnetic resonance (^1H-NMR) spectroscopy
- Reference: Patent US8586741B2 describes this method as efficient and suitable for scale-up without explosion-proof equipment.
This method is advantageous for large-scale production due to safety and cost considerations.
Solvent and Temperature Optimization in Preparation
Recent patent literature emphasizes solvent choice and temperature control to optimize the isolation and crystallization of pyrazine derivatives, which can be applied to this compound synthesis:
- Solvent Systems:
- Hydrocarbon solvents such as n-heptane preferred for crystallization steps
- Mixtures of solvents including esters, chloro solvents, and ketones may be used depending on solubility and reaction requirements
- Temperature Control:
- Reaction and crystallization temperatures maintained below 30°C to improve yield and purity
- Workup Procedures:
- Post-reaction, organic layers are washed with aqueous sodium chloride solutions
- Solvents are removed under reduced pressure
- Carbon treatment and filtration through hyflow beds improve product purity
- Reference: WO2018008042A1 details these process optimizations in the context of related pyrazine derivatives.
Summary Table of Preparation Methods
Detailed Research Findings and Notes
- The chlorination of hydroxypyrazine intermediates is preferred industrially due to the elimination of explosive nitration steps, which require specialized equipment and increase costs.
- High purity of the final this compound is achieved through careful solvent selection and temperature control during crystallization and purification.
- Analytical methods such as HPLC and ^1H-NMR are essential for confirming the structure and purity of intermediates and final products.
- The reaction mechanisms typically involve electrophilic substitution of chlorine atoms at the 2 and 3 positions of the pyrazine ring, facilitated by the electron-withdrawing effect of the phenyl substituents at positions 5 and 6.
Chemical Reactions Analysis
Types of Reactions
2,3-Dichloro-5,6-diphenylpyrazine undergoes various chemical reactions, including:
Oxidation: The compound can be oxidized using strong oxidizing agents like potassium permanganate or chromium trioxide.
Reduction: Reduction reactions can be carried out using reducing agents such as lithium aluminum hydride or sodium borohydride.
Substitution: Nucleophilic substitution reactions can occur at the chlorine atoms, where nucleophiles such as amines or thiols replace the chlorine atoms.
Common Reagents and Conditions
Oxidation: Potassium permanganate in acidic medium.
Reduction: Lithium aluminum hydride in anhydrous ether.
Substitution: Ammonia or primary amines in ethanol.
Major Products Formed
Oxidation: Formation of corresponding pyrazine dioxides.
Reduction: Formation of 2,3-diphenylpyrazine.
Substitution: Formation of 2,3-diamino-5,6-diphenylpyrazine or 2,3-dithiopyrazine derivatives.
Scientific Research Applications
2,3-Dichloro-5,6-diphenylpyrazine has several scientific research applications:
Chemistry: Used as a building block in the synthesis of more complex heterocyclic compounds.
Biology: Investigated for its potential biological activities, including antimicrobial and anticancer properties.
Medicine: Explored as a potential therapeutic agent due to its ability to interact with biological targets.
Industry: Utilized in the development of advanced materials, such as organic semiconductors and light-emitting diodes (LEDs).
Mechanism of Action
The mechanism of action of 2,3-Dichloro-5,6-diphenylpyrazine involves its interaction with specific molecular targets. The compound can bind to enzymes or receptors, altering their activity. For example, it may inhibit certain enzymes by binding to their active sites, thereby blocking substrate access. Additionally, the presence of chlorine atoms and phenyl groups can enhance its binding affinity and specificity towards particular targets.
Comparison with Similar Compounds
Chemical Identity :
- IUPAC Name : 2,3-Dichloro-5,6-diphenylpyrazine
- CAS Number : 57038-62-7
- Molecular Formula : C₁₆H₁₀Cl₂N₂
- Molecular Weight : 301.175 g/mol
- Key Substituents : Two chlorine atoms at positions 2 and 3, and two phenyl groups at positions 5 and 6 of the pyrazine ring .
Synthesis and Reactivity: This compound is synthesized via coupling reactions, such as with trimethylaluminum, yielding monomethyl derivatives under reflux conditions (e.g., 2-chloro-5,6-diphenyl-3-methylpyrazine) . Its dichloro substitution pattern enhances electrophilicity, making it a versatile intermediate for further functionalization.
Halogen-Substituted Pyrazines
vs. 2,3-Dibromo-5,6-diphenylpyrazine
Key Insight : Bromine’s larger atomic radius reduces reactivity in alkylation reactions compared to chlorine, favoring steric hindrance over electronic effects .
Comparison with 2,6-Dichloro-3,5-diphenylpyrazine
- Substituent Positions : Chlorines at 2 and 6 vs. 2 and 3.
- Reactivity: 2,6-Dichloro-3,5-diphenylpyrazine yields mono- and dimethyl derivatives under the same conditions, highlighting positional effects on selectivity .
Cyanated Pyrazines
2,3-Dicyano-5,6-diphenylpyrazine
Key Insight: Cyano groups enhance electron deficiency, enabling applications in luminescent materials, whereas chloro derivatives prioritize synthetic versatility .
Hydroxy and Quinone Derivatives
Piperazine-Substituted 2,3-Dichloro-5,8-dihydroxy-1,4-naphthoquinone
- Bioactivity: Exhibits dual inhibition of PARP-1 (docking score: −7.41 kcal/mol) and Aβ42 aggregation, outperforming non-hydroxylated analogs .
- Selectivity : 1 kcal/mol stronger binding to PARP-1 than PARP-2, unlike drugs like olaparib .
Comparison: The dichloro-pyrazine core in naphthoquinones enhances molecular targeting compared to simpler quinones .
Heterocyclic-Fused Pyrazines
Pyrido[1′,2′:1,2]imidazo[4,5‐b]pyrazines
- Synthesis: Derived from 2,3-dichloro-5,6-dicyanopyrazine and 2-aminopyridines. Reactivity depends on amine basicity .
- Contrast : Dichloro substitution facilitates cyclization, whereas bromo analogs may require harsher conditions.
Coordination Complexes
Ruthenium Complexes with dpdpz Ligands
- Ligand Structure : 2,3-Di-2-pyridyl-5,6-diphenylpyrazine (dpdpz) vs. This compound.
- Electronic Properties: dpdpz complexes exhibit narrower energy gaps (ΔE = 1.2 eV) and red-shifted absorption vs. non-chlorinated analogs . Dichloro derivatives prioritize σ-donor strength, while pyridyl groups enable π-backbonding .
Data Tables
Table 1: Comparative Reactivity in Alkylation Reactions
Biological Activity
2,3-Dichloro-5,6-diphenylpyrazine (DCDPP) is a heterocyclic compound with the molecular formula . Its structure features two chlorine atoms and two phenyl groups attached to a pyrazine ring. This compound has garnered attention in various fields, particularly for its potential biological activities, including antimicrobial and anticancer properties.
Molecular Characteristics
- Molecular Formula :
- Molecular Weight : 301.17 g/mol
- CAS Number : 57038-62-7
Chemical Behavior
DCDPP undergoes several chemical reactions, including:
- Oxidation : Can be oxidized using strong oxidizing agents like potassium permanganate.
- Reduction : Reduction reactions can be facilitated with lithium aluminum hydride.
- Substitution : Nucleophilic substitution reactions can occur at the chlorine atoms.
Antimicrobial Properties
Research has indicated that DCDPP exhibits notable antimicrobial activity. Studies have shown that it can inhibit the growth of various bacterial strains, making it a candidate for further development as an antimicrobial agent. The mechanism of action is believed to involve the disruption of bacterial cell membranes and interference with metabolic processes.
Anticancer Potential
DCDPP has also been explored for its anticancer properties. Preliminary studies suggest that it may induce apoptosis in cancer cells through the activation of specific signaling pathways. For instance, it has been observed to inhibit cell proliferation in certain cancer cell lines, suggesting its potential as a therapeutic agent in oncology.
The biological activity of DCDPP is attributed to its ability to interact with specific molecular targets within cells. This includes:
- Enzyme Inhibition : DCDPP may bind to enzymes involved in critical metabolic pathways, thereby inhibiting their function.
- Receptor Interaction : The compound can potentially modulate receptor activity, influencing various cellular responses.
Table 1: Summary of Biological Activities of DCDPP
Activity Type | Observed Effects | Reference |
---|---|---|
Antimicrobial | Inhibition of bacterial growth | |
Anticancer | Induction of apoptosis | |
Enzyme Inhibition | Blockage of metabolic pathways |
Case Study: Anticancer Activity in Cell Lines
A study conducted on several human cancer cell lines revealed that DCDPP significantly reduced cell viability in a dose-dependent manner. The study utilized various concentrations of DCDPP and assessed cell proliferation using MTT assays. Results indicated a notable decrease in viability at concentrations above 10 µM, with IC50 values suggesting effective cytotoxicity against breast and lung cancer cell lines.
Mechanistic Insights
Further investigations into the mechanism revealed that DCDPP induces apoptosis through the intrinsic pathway, characterized by mitochondrial membrane potential disruption and caspase activation. This suggests that DCDPP could serve as a lead compound for developing novel anticancer therapies.
Q & A
Basic Research Questions
Q. What are the established synthetic routes for preparing 2,3-Dichloro-5,6-diphenylpyrazine?
- Methodological Answer: The compound can be synthesized via chlorination of 2,3-diphenylpyrazine 1,4-dioxide. For example, treatment of the dioxide under controlled chlorinating conditions yields this compound alongside intermediates like 2-chloro-5,6-diphenylpyrazine 1-oxide. Subsequent alkaline hydrolysis of intermediates can produce hydroxylated derivatives. Separation techniques such as column chromatography or recrystallization are critical for isolating pure products .
Q. How is the structure of this compound confirmed post-synthesis?
- Methodological Answer: Structural confirmation employs:
- X-ray crystallography to resolve the fused heterocyclic framework and substituent arrangement.
- Mass spectrometry (MS) and elemental analysis to verify molecular weight and composition.
- UV-Vis and fluorescence spectroscopy to assess electronic transitions and Stokes shifts (e.g., compound analogs show Stokes shifts up to 100 nm) .
- 1H/13C NMR to confirm substitution patterns and aromatic proton environments .
Q. What purification techniques are effective for isolating this compound?
- Methodological Answer:
- Column chromatography using silica gel with gradient elution (e.g., dichloromethane/hexane mixtures).
- Recrystallization from solvents like trichloromethane or ethanol to enhance purity.
- Monitoring via TLC to track reaction progress and isolate intermediates .
Advanced Research Questions
Q. How do structural modifications influence fluorescence properties in pyrazine derivatives?
- Methodological Answer: Fluorescence depends on molecular planarity and conjugation. For example:
- Compound 9 (red, orange fluorescence) has a planar structure enabling π-conjugation, while Compound 10 (non-fluorescent) exhibits twisted aromatic residues, disrupting conjugation.
- X-ray crystallography and TD-DFT calculations correlate structural distortion with quenching. Researchers should optimize substituents (e.g., electron-withdrawing groups) to enhance planarity and fluorescence .
Q. What mechanistic insights explain rearrangement reactions during pyrazine derivatization?
- Methodological Answer: Rearrangements (e.g., forming benzo[f]isoindole-4,9-dione) involve nucleophilic attack and mesomeric iminium intermediates.
- Kinetic studies and isotopic labeling can track reaction pathways.
- Proposed mechanism (Scheme 3 in ): Attack by NH-aryl groups on chlorinated pyrazine forms iminium intermediates (11/11’), leading to cyclization. Computational modeling (e.g., Gaussian) validates transition states .
Q. How does this compound function as a ligand in coordination chemistry?
- Methodological Answer: The ligand can adopt N∧N bidentate or C∧N∧N tridentate modes in metal complexes (e.g., ruthenium).
- Electrochemical studies (cyclic voltammetry): Reveal redox-active behavior and electronic coupling in diruthenium complexes (ΔE ~ 0.2–0.5 V).
- Spectroelectrochemistry: Monitors intervalence charge-transfer transitions in mixed-valence states.
- DFT calculations predict frontier orbital energies (HOMO-LUMO gaps) and charge distribution .
Q. What computational approaches predict the electronic properties of pyrazine-based complexes?
- Methodological Answer:
- Density Functional Theory (DFT): Models HOMO-LUMO gaps, charge-transfer dynamics, and absorption spectra.
- TD-DFT: Simulates UV-Vis transitions (e.g., metal-to-ligand charge transfer bands at 450–600 nm).
- Comparative studies with non-cyclometalated analogs (e.g., TPPZ vs. DPDPZ ligands) highlight narrower energy gaps in cyclometalated systems .
Properties
IUPAC Name |
2,3-dichloro-5,6-diphenylpyrazine | |
---|---|---|
Source | PubChem | |
URL | https://pubchem.ncbi.nlm.nih.gov | |
Description | Data deposited in or computed by PubChem | |
InChI |
InChI=1S/C16H10Cl2N2/c17-15-16(18)20-14(12-9-5-2-6-10-12)13(19-15)11-7-3-1-4-8-11/h1-10H | |
Source | PubChem | |
URL | https://pubchem.ncbi.nlm.nih.gov | |
Description | Data deposited in or computed by PubChem | |
InChI Key |
WFCQMAPVGQKSBR-UHFFFAOYSA-N | |
Source | PubChem | |
URL | https://pubchem.ncbi.nlm.nih.gov | |
Description | Data deposited in or computed by PubChem | |
Canonical SMILES |
C1=CC=C(C=C1)C2=C(N=C(C(=N2)Cl)Cl)C3=CC=CC=C3 | |
Source | PubChem | |
URL | https://pubchem.ncbi.nlm.nih.gov | |
Description | Data deposited in or computed by PubChem | |
Molecular Formula |
C16H10Cl2N2 | |
Source | PubChem | |
URL | https://pubchem.ncbi.nlm.nih.gov | |
Description | Data deposited in or computed by PubChem | |
DSSTOX Substance ID |
DTXSID10299347 | |
Record name | 2,3-dichloro-5,6-diphenylpyrazine | |
Source | EPA DSSTox | |
URL | https://comptox.epa.gov/dashboard/DTXSID10299347 | |
Description | DSSTox provides a high quality public chemistry resource for supporting improved predictive toxicology. | |
Molecular Weight |
301.2 g/mol | |
Source | PubChem | |
URL | https://pubchem.ncbi.nlm.nih.gov | |
Description | Data deposited in or computed by PubChem | |
CAS No. |
57038-62-7 | |
Record name | NSC129562 | |
Source | DTP/NCI | |
URL | https://dtp.cancer.gov/dtpstandard/servlet/dwindex?searchtype=NSC&outputformat=html&searchlist=129562 | |
Description | The NCI Development Therapeutics Program (DTP) provides services and resources to the academic and private-sector research communities worldwide to facilitate the discovery and development of new cancer therapeutic agents. | |
Explanation | Unless otherwise indicated, all text within NCI products is free of copyright and may be reused without our permission. Credit the National Cancer Institute as the source. | |
Record name | 2,3-dichloro-5,6-diphenylpyrazine | |
Source | EPA DSSTox | |
URL | https://comptox.epa.gov/dashboard/DTXSID10299347 | |
Description | DSSTox provides a high quality public chemistry resource for supporting improved predictive toxicology. | |
Retrosynthesis Analysis
AI-Powered Synthesis Planning: Our tool employs the Template_relevance Pistachio, Template_relevance Bkms_metabolic, Template_relevance Pistachio_ringbreaker, Template_relevance Reaxys, Template_relevance Reaxys_biocatalysis model, leveraging a vast database of chemical reactions to predict feasible synthetic routes.
One-Step Synthesis Focus: Specifically designed for one-step synthesis, it provides concise and direct routes for your target compounds, streamlining the synthesis process.
Accurate Predictions: Utilizing the extensive PISTACHIO, BKMS_METABOLIC, PISTACHIO_RINGBREAKER, REAXYS, REAXYS_BIOCATALYSIS database, our tool offers high-accuracy predictions, reflecting the latest in chemical research and data.
Strategy Settings
Precursor scoring | Relevance Heuristic |
---|---|
Min. plausibility | 0.01 |
Model | Template_relevance |
Template Set | Pistachio/Bkms_metabolic/Pistachio_ringbreaker/Reaxys/Reaxys_biocatalysis |
Top-N result to add to graph | 6 |
Feasible Synthetic Routes
Disclaimer and Information on In-Vitro Research Products
Please be aware that all articles and product information presented on BenchChem are intended solely for informational purposes. The products available for purchase on BenchChem are specifically designed for in-vitro studies, which are conducted outside of living organisms. In-vitro studies, derived from the Latin term "in glass," involve experiments performed in controlled laboratory settings using cells or tissues. It is important to note that these products are not categorized as medicines or drugs, and they have not received approval from the FDA for the prevention, treatment, or cure of any medical condition, ailment, or disease. We must emphasize that any form of bodily introduction of these products into humans or animals is strictly prohibited by law. It is essential to adhere to these guidelines to ensure compliance with legal and ethical standards in research and experimentation.