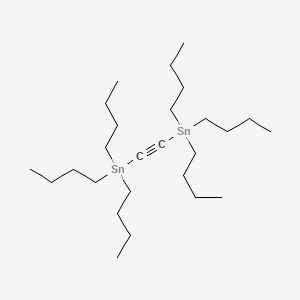
Bis(tributylstannyl)acetylene
Overview
Description
Bis(tributylstannyl)acetylene: is an organotin compound with the molecular formula C26H54Sn2 . It is a pale yellow liquid that is primarily used as a reagent in organic synthesis. The compound is known for its utility in forming carbon-carbon bonds and is often employed in the synthesis of complex organic molecules.
Mechanism of Action
Target of Action
Bis(tributylstannyl)acetylene is a chemical compound used in various chemical reactions, particularly in the field of organic synthesis . The primary targets of this compound are the reactant molecules in these chemical reactions .
Mode of Action
This compound interacts with its targets through a process known as the Stille coupling . This is a type of cross-coupling reaction that involves the reaction of an organotin compound, such as this compound, with an organic halide in the presence of a palladium catalyst .
Biochemical Pathways
The Stille coupling reaction involving this compound can lead to the formation of various organic compounds . These compounds can then participate in other biochemical pathways, depending on their specific chemical structures .
Result of Action
The result of this compound’s action is the formation of new organic compounds through the Stille coupling reaction . These compounds can have various applications, depending on their specific chemical structures .
Preparation Methods
Synthetic Routes and Reaction Conditions: The preparation of bis(tributylstannyl)acetylene typically involves the reaction of lithium acetylide-ethylenediamine complex with tributyltin chloride in the presence of tetrahydrofuran. The reaction is carried out under nitrogen atmosphere to prevent oxidation. The mixture is stirred at room temperature for an extended period, followed by hydrolysis and purification steps to obtain the desired product .
Industrial Production Methods: Industrial production of this compound follows similar synthetic routes but on a larger scale. The process involves stringent control of reaction conditions to ensure high yield and purity. The use of automated systems for addition of reagents and temperature control is common in industrial settings to maintain consistency and safety.
Chemical Reactions Analysis
Types of Reactions: Bis(tributylstannyl)acetylene undergoes various types of chemical reactions, including:
Oxidation: The compound can be oxidized to form stannic derivatives.
Reduction: It can be reduced to form simpler organotin compounds.
Substitution: The stannyl groups can be substituted with other functional groups through reactions with appropriate reagents.
Common Reagents and Conditions:
Oxidation: Reagents such as hydrogen peroxide or other oxidizing agents.
Reduction: Reagents like lithium aluminum hydride.
Substitution: Palladium-catalyzed coupling reactions are common, using reagents like tetrakis(triphenylphosphine)palladium(0).
Major Products: The major products formed from these reactions depend on the specific conditions and reagents used. For example, oxidation may yield stannic oxides, while substitution reactions can produce a variety of functionalized organic compounds.
Scientific Research Applications
Chemistry: Bis(tributylstannyl)acetylene is widely used in organic synthesis for forming carbon-carbon bonds. It is particularly useful in the synthesis of complex organic molecules and natural products.
Biology and Medicine: In biological research, the compound is used as a precursor for the synthesis of bioactive molecules. Its ability to form stable carbon-carbon bonds makes it valuable in the development of pharmaceuticals.
Industry: In industrial applications, this compound is used in the production of polymers and other advanced materials. Its role as a reagent in the synthesis of specialty chemicals is also significant.
Comparison with Similar Compounds
- Bis(trimethylstannyl)acetylene
- Bis(triphenylstannyl)acetylene
- Bis(tributyltin)acetylene
Comparison: Bis(tributylstannyl)acetylene is unique due to its specific reactivity and stability. Compared to bis(trimethylstannyl)acetylene, it offers better solubility in organic solvents. Compared to bis(triphenylstannyl)acetylene, it provides a more favorable reactivity profile for certain types of coupling reactions. Its stability and ease of handling make it a preferred choice in many synthetic applications.
Properties
IUPAC Name |
tributyl(2-tributylstannylethynyl)stannane | |
---|---|---|
Source | PubChem | |
URL | https://pubchem.ncbi.nlm.nih.gov | |
Description | Data deposited in or computed by PubChem | |
InChI |
InChI=1S/6C4H9.C2.2Sn/c6*1-3-4-2;1-2;;/h6*1,3-4H2,2H3;;; | |
Source | PubChem | |
URL | https://pubchem.ncbi.nlm.nih.gov | |
Description | Data deposited in or computed by PubChem | |
InChI Key |
CAUONNQGFXOEMY-UHFFFAOYSA-N | |
Source | PubChem | |
URL | https://pubchem.ncbi.nlm.nih.gov | |
Description | Data deposited in or computed by PubChem | |
Canonical SMILES |
CCCC[Sn](CCCC)(CCCC)C#C[Sn](CCCC)(CCCC)CCCC | |
Source | PubChem | |
URL | https://pubchem.ncbi.nlm.nih.gov | |
Description | Data deposited in or computed by PubChem | |
Molecular Formula |
C26H54Sn2 | |
Source | PubChem | |
URL | https://pubchem.ncbi.nlm.nih.gov | |
Description | Data deposited in or computed by PubChem | |
DSSTOX Substance ID |
DTXSID10308347 | |
Record name | Bis(tributylstannyl)acetylene | |
Source | EPA DSSTox | |
URL | https://comptox.epa.gov/dashboard/DTXSID10308347 | |
Description | DSSTox provides a high quality public chemistry resource for supporting improved predictive toxicology. | |
Molecular Weight |
604.1 g/mol | |
Source | PubChem | |
URL | https://pubchem.ncbi.nlm.nih.gov | |
Description | Data deposited in or computed by PubChem | |
CAS No. |
994-71-8 | |
Record name | 994-71-8 | |
Source | DTP/NCI | |
URL | https://dtp.cancer.gov/dtpstandard/servlet/dwindex?searchtype=NSC&outputformat=html&searchlist=203205 | |
Description | The NCI Development Therapeutics Program (DTP) provides services and resources to the academic and private-sector research communities worldwide to facilitate the discovery and development of new cancer therapeutic agents. | |
Explanation | Unless otherwise indicated, all text within NCI products is free of copyright and may be reused without our permission. Credit the National Cancer Institute as the source. | |
Record name | Bis(tributylstannyl)acetylene | |
Source | EPA DSSTox | |
URL | https://comptox.epa.gov/dashboard/DTXSID10308347 | |
Description | DSSTox provides a high quality public chemistry resource for supporting improved predictive toxicology. | |
Record name | Bis(tributylstannyl)acetylene | |
Source | European Chemicals Agency (ECHA) | |
URL | https://echa.europa.eu/information-on-chemicals | |
Description | The European Chemicals Agency (ECHA) is an agency of the European Union which is the driving force among regulatory authorities in implementing the EU's groundbreaking chemicals legislation for the benefit of human health and the environment as well as for innovation and competitiveness. | |
Explanation | Use of the information, documents and data from the ECHA website is subject to the terms and conditions of this Legal Notice, and subject to other binding limitations provided for under applicable law, the information, documents and data made available on the ECHA website may be reproduced, distributed and/or used, totally or in part, for non-commercial purposes provided that ECHA is acknowledged as the source: "Source: European Chemicals Agency, http://echa.europa.eu/". Such acknowledgement must be included in each copy of the material. ECHA permits and encourages organisations and individuals to create links to the ECHA website under the following cumulative conditions: Links can only be made to webpages that provide a link to the Legal Notice page. | |
Synthesis routes and methods I
Procedure details
Synthesis routes and methods II
Procedure details
Retrosynthesis Analysis
AI-Powered Synthesis Planning: Our tool employs the Template_relevance Pistachio, Template_relevance Bkms_metabolic, Template_relevance Pistachio_ringbreaker, Template_relevance Reaxys, Template_relevance Reaxys_biocatalysis model, leveraging a vast database of chemical reactions to predict feasible synthetic routes.
One-Step Synthesis Focus: Specifically designed for one-step synthesis, it provides concise and direct routes for your target compounds, streamlining the synthesis process.
Accurate Predictions: Utilizing the extensive PISTACHIO, BKMS_METABOLIC, PISTACHIO_RINGBREAKER, REAXYS, REAXYS_BIOCATALYSIS database, our tool offers high-accuracy predictions, reflecting the latest in chemical research and data.
Strategy Settings
Precursor scoring | Relevance Heuristic |
---|---|
Min. plausibility | 0.01 |
Model | Template_relevance |
Template Set | Pistachio/Bkms_metabolic/Pistachio_ringbreaker/Reaxys/Reaxys_biocatalysis |
Top-N result to add to graph | 6 |
Feasible Synthetic Routes
Q1: What is the primary application of Bis(tributylstannyl)acetylene in organic synthesis?
A1: this compound serves as a valuable reagent in palladium-catalyzed Stille coupling reactions. [, , , ] It readily reacts with aryl halides, enabling the efficient synthesis of symmetrical diarylalkynes, crucial building blocks in organic synthesis, particularly for constructing conjugated polymers and oligomers. [, , ]
Q2: Can you provide examples of specific compounds synthesized using this compound and their potential applications?
A2: Researchers have successfully utilized this compound to synthesize:
- Oligomers with recurring benzodiazaborole units: These oligomers exhibit promising optical and electrochemical properties due to their expanded electron systems, potentially leading to applications in optoelectronic devices. []
- Pyromellitic Diimide-Ethynylene-Based Homopolymers: These polymers demonstrate n-channel semiconducting activity, offering new avenues for developing n-channel organic field-effect transistors (OFETs). []
- Naphthalenetetracarboxylic diimide (NDI) containing materials: These materials are explored for their potential in organic electronics and supramolecular chemistry. []
Q3: How does the Stille coupling reaction involving this compound proceed?
A3: In the presence of a palladium catalyst, this compound undergoes a transmetalation reaction with an aryl halide, replacing one tributylstannyl group with the aryl group. Subsequent reductive elimination yields the desired diarylalkyne, regenerating the palladium catalyst. [, , , ]
Q4: What are the structural characteristics of this compound?
A4: While the provided research papers do not explicitly state the molecular formula and weight, based on its chemical name and structure, this compound likely possesses the following:
Q5: Are there any concerns regarding the stability or compatibility of this compound?
A6: Although the provided research doesn't delve into specific stability details, organotin compounds, including this compound, are generally sensitive to air and moisture. [, ] Therefore, handling them under inert atmospheres, such as nitrogen or argon, is crucial to prevent degradation.
Q6: What analytical techniques are employed to characterize compounds synthesized using this compound?
A6: Researchers commonly employ various spectroscopic and analytical techniques to characterize the products, including:
- Nuclear Magnetic Resonance (NMR) Spectroscopy: For structural elucidation and confirming the formation of desired bonds. [, , ]
- UV-Visible Spectroscopy: To investigate the electronic properties and conjugation length of the synthesized compounds. [, ]
- X-ray Diffraction (XRD): To analyze the solid-state structure and packing of the materials. []
- Atomic Force Microscopy (AFM): To study surface morphology and film formation. []
- Cyclic Voltammetry: To investigate electrochemical properties and energy levels. []
Disclaimer and Information on In-Vitro Research Products
Please be aware that all articles and product information presented on BenchChem are intended solely for informational purposes. The products available for purchase on BenchChem are specifically designed for in-vitro studies, which are conducted outside of living organisms. In-vitro studies, derived from the Latin term "in glass," involve experiments performed in controlled laboratory settings using cells or tissues. It is important to note that these products are not categorized as medicines or drugs, and they have not received approval from the FDA for the prevention, treatment, or cure of any medical condition, ailment, or disease. We must emphasize that any form of bodily introduction of these products into humans or animals is strictly prohibited by law. It is essential to adhere to these guidelines to ensure compliance with legal and ethical standards in research and experimentation.