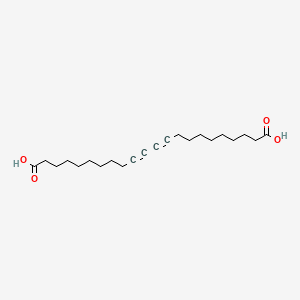
10,12-Docosadiynedioic acid
Overview
Description
10,12-Docosadiynedioic acid is a long-chain diacetylenic diacid with the molecular formula C22H34O4. It is characterized by the presence of two triple bonds at the 10th and 12th positions of the docosanoic acid chain. This compound is known for its unique polymerizable properties, making it valuable in various scientific and industrial applications .
Mechanism of Action
Mode of Action
The exact mode of action of 10,12-Docosadiynedioic acid is currently unknown. It is known that the compound is involved in the synthesis of dimesogenic liquid crystals . The exact interaction of this compound with its targets and the resulting changes are subjects of ongoing research.
Biochemical Pathways
This compound is involved in the synthesis of dimesogenic liquid crystals . These liquid crystals are formed by the esterification of this compound with both cholesterol and azobenzene groups
Result of Action
It is known that the compound plays a role in the synthesis of dimesogenic liquid crystals .
Action Environment
The action, efficacy, and stability of this compound can be influenced by various environmental factors. For instance, the compound is light-sensitive and should be stored in a cool place, away from strong oxidizing agents
Preparation Methods
Synthetic Routes and Reaction Conditions
10,12-Docosadiynedioic acid can be synthesized through a multi-step process involving the coupling of appropriate alkyne precursors. One common method involves the oxidative coupling of terminal alkynes using catalysts such as copper(I) chloride and palladium(II) acetate. The reaction is typically carried out in an inert atmosphere to prevent unwanted side reactions .
Industrial Production Methods
Industrial production of this compound often involves the esterification of intermediate compounds followed by hydrolysis to yield the final diacid. The process requires precise control of reaction conditions, including temperature, pressure, and pH, to ensure high yield and purity .
Chemical Reactions Analysis
Types of Reactions
10,12-Docosadiynedioic acid undergoes various chemical reactions, including:
Oxidation: The triple bonds can be oxidized to form diketones or carboxylic acids.
Reduction: Reduction of the triple bonds can yield alkenes or alkanes.
Substitution: The carboxylic acid groups can participate in esterification or amidation reactions.
Common Reagents and Conditions
Oxidation: Potassium permanganate or osmium tetroxide in the presence of a suitable solvent.
Reduction: Hydrogen gas with a palladium or platinum catalyst.
Substitution: Alcohols or amines in the presence of a dehydrating agent such as dicyclohexylcarbodiimide.
Major Products Formed
Oxidation: Formation of diketones or carboxylic acids.
Reduction: Formation of alkenes or alkanes.
Substitution: Formation of esters or amides.
Scientific Research Applications
Polymer Chemistry
DDA serves as a polymerizable diacid, primarily used in the synthesis of advanced materials. Its ability to form liquid crystals through esterification reactions has been demonstrated. For instance, DDA has been utilized to synthesize dimesogenic liquid crystals that incorporate cholesterol and azobenzene groups, which are critical for developing responsive materials in photonics and display technologies .
Case Study: Liquid Crystal Synthesis
In a study focused on the synthesis of dimesogenic liquid crystals, DDA was esterified to create materials that exhibit unique optical properties. The incorporation of DDA into these systems enhances their thermal stability and responsiveness to external stimuli, making them suitable for applications in smart coatings and displays.
Supramolecular Chemistry
DDA has been investigated for its role in forming supramolecular structures, particularly hydrogels. Research indicates that DDA can gelate water when combined with amino acids like arginine, leading to the formation of fibrous nanostructures. This property is attributed to the guanidinium-carboxylate interactions that stabilize the fibrous network .
Case Study: Hydrogel Formation
A study demonstrated that a hydrogel formed from DDA and arginine exhibited significant structural integrity upon UV irradiation, which induced polymerization of the diacetylene groups. The resulting nanofibers displayed a color change from white to orange, indicating successful oligomerization while maintaining chirality . This property is advantageous for applications in drug delivery systems and tissue engineering.
Biomedical Applications
DDA's chemical structure allows it to be utilized as a building block for pharmaceutical compounds. Its derivatives have shown potential anti-inflammatory and analgesic properties, making them candidates for drug development .
Example: Drug Delivery Systems
Research has explored using DDA-based hydrogels as drug delivery vehicles. These systems can encapsulate therapeutic agents and release them in a controlled manner, improving efficacy and reducing side effects.
Nanotechnology
In nanotechnology, DDA's ability to form organized structures at the nanoscale is leveraged for creating advanced materials with specific functionalities. The self-assembly of DDA into nanofibers can be utilized in sensors and electronic devices.
Case Study: Sensor Development
Recent advancements have seen the use of DDA-derived nanostructures in developing color-changing sensors for volatile organic compounds (VOCs). These sensors exploit the optical properties of DDA when subjected to environmental changes, providing a novel approach for detecting pollutants .
Comparison with Similar Compounds
Similar Compounds
- 9,11-Docosadiynedioic acid
- 8,10-Docosadiynedioic acid
- 7,9-Docosadiynedioic acid
Uniqueness
10,12-Docosadiynedioic acid is unique due to its specific positioning of triple bonds, which allows for efficient polymerization and the formation of stable polymer networks. This makes it particularly valuable in applications requiring photoresponsive materials and advanced coatings .
Biological Activity
10,12-Docosadiynedioic acid (DDA) is a unique dicarboxylic acid characterized by its conjugated triple bonds and dicarboxylic functionality. Its molecular formula is , with a molecular weight of 362.51 g/mol. This compound has garnered attention in various fields, including materials science and biochemistry, due to its distinctive properties and potential applications.
Chemical Identifiers :
Property | Value |
---|---|
CAS Number | 28393-02-4 |
Molecular Formula | C22H34O4 |
Molecular Weight | 362.51 g/mol |
Melting Point | 110-112 °C |
Boiling Point | 566.7 °C at 760 mmHg |
Density | 1.043 g/cm³ |
DDA is insoluble in water and is sensitive to light, necessitating careful storage conditions to maintain its stability .
Supramolecular Structures
Recent studies have demonstrated that DDA can form supramolecular structures when combined with biological molecules such as L-arginine. The formation of a hydrogel from DDA and L-arginine was observed, which exhibited fibrous structures upon gelation. This hydrogel showed significant optical properties and was confirmed through various spectroscopic techniques, including nuclear magnetic resonance (NMR) and X-ray diffraction (XRD) analyses .
Key Findings :
- The interaction between DDA and L-arginine leads to the formation of a fibrous hydrogel.
- Upon UV irradiation, the hydrogel polymerizes into diacetylene oligomers, resulting in a color change from white to orange.
- The chirality of L-arginine was found to influence the structural properties of the resulting oligomers, indicating potential applications in chiral materials .
Polymerization and Gelation
DDA's ability to undergo polymerization under UV light suggests its utility in creating functional materials with specific mechanical and optical properties. The polymerization process involves the transformation of the diacetylene groups into more complex structures while maintaining the fibrous morphology of the hydrogel .
Study on Hydrogel Formation
In an experimental study, researchers synthesized a DDA–arginine hydrogel by dissolving DDA in an aqueous arginine solution. The resultant gel exhibited unique properties:
- Gelation Time : The gel formed after 24 hours at room temperature.
- Microscopic Analysis : Scanning electron microscopy revealed fibrous structures ranging from 10 to 40 nm in width.
The study concluded that the guanidinium–carboxylate interaction was crucial for the formation of these fibrous structures and that UV irradiation led to significant changes in the material's properties .
Applications
The unique properties of DDA make it suitable for various applications, including:
- Material Science : As a precursor for dimesogenic liquid crystals due to its polymerizable diacid nature.
- Biotechnology : Potential use in drug delivery systems or as scaffolding materials in tissue engineering due to its biocompatibility when combined with amino acids like arginine.
Properties
IUPAC Name |
docosa-10,12-diynedioic acid | |
---|---|---|
Source | PubChem | |
URL | https://pubchem.ncbi.nlm.nih.gov | |
Description | Data deposited in or computed by PubChem | |
InChI |
InChI=1S/C22H34O4/c23-21(24)19-17-15-13-11-9-7-5-3-1-2-4-6-8-10-12-14-16-18-20-22(25)26/h5-20H2,(H,23,24)(H,25,26) | |
Source | PubChem | |
URL | https://pubchem.ncbi.nlm.nih.gov | |
Description | Data deposited in or computed by PubChem | |
InChI Key |
XCUAGNLOPZWTEH-UHFFFAOYSA-N | |
Source | PubChem | |
URL | https://pubchem.ncbi.nlm.nih.gov | |
Description | Data deposited in or computed by PubChem | |
Canonical SMILES |
C(CCCCC(=O)O)CCCC#CC#CCCCCCCCCC(=O)O | |
Source | PubChem | |
URL | https://pubchem.ncbi.nlm.nih.gov | |
Description | Data deposited in or computed by PubChem | |
Molecular Formula |
C22H34O4 | |
Source | PubChem | |
URL | https://pubchem.ncbi.nlm.nih.gov | |
Description | Data deposited in or computed by PubChem | |
DSSTOX Substance ID |
DTXSID20337704 | |
Record name | 10,12-Docosadiynedioic acid | |
Source | EPA DSSTox | |
URL | https://comptox.epa.gov/dashboard/DTXSID20337704 | |
Description | DSSTox provides a high quality public chemistry resource for supporting improved predictive toxicology. | |
Molecular Weight |
362.5 g/mol | |
Source | PubChem | |
URL | https://pubchem.ncbi.nlm.nih.gov | |
Description | Data deposited in or computed by PubChem | |
CAS No. |
28393-02-4 | |
Record name | 10,12-Docosadiynedioic acid | |
Source | EPA DSSTox | |
URL | https://comptox.epa.gov/dashboard/DTXSID20337704 | |
Description | DSSTox provides a high quality public chemistry resource for supporting improved predictive toxicology. | |
Retrosynthesis Analysis
AI-Powered Synthesis Planning: Our tool employs the Template_relevance Pistachio, Template_relevance Bkms_metabolic, Template_relevance Pistachio_ringbreaker, Template_relevance Reaxys, Template_relevance Reaxys_biocatalysis model, leveraging a vast database of chemical reactions to predict feasible synthetic routes.
One-Step Synthesis Focus: Specifically designed for one-step synthesis, it provides concise and direct routes for your target compounds, streamlining the synthesis process.
Accurate Predictions: Utilizing the extensive PISTACHIO, BKMS_METABOLIC, PISTACHIO_RINGBREAKER, REAXYS, REAXYS_BIOCATALYSIS database, our tool offers high-accuracy predictions, reflecting the latest in chemical research and data.
Strategy Settings
Precursor scoring | Relevance Heuristic |
---|---|
Min. plausibility | 0.01 |
Model | Template_relevance |
Template Set | Pistachio/Bkms_metabolic/Pistachio_ringbreaker/Reaxys/Reaxys_biocatalysis |
Top-N result to add to graph | 6 |
Feasible Synthetic Routes
Q1: What is the structural characterization of 10,12-Docosadiynedioic acid?
A: While the provided research articles don't explicitly state the molecular formula and weight of this compound, they describe its use as a building block for synthesizing di-mesogenic liquid crystals. These crystals are formed by esterifying this compound with cholesterol and 4-alkyl-4′-hydroxyazobenzene (or 4-hydroxyazobenzene) [, ]. This suggests the presence of two carboxylic acid groups in its structure, capable of forming ester bonds. Unfortunately, the articles lack detailed spectroscopic data for this compound.
Q2: How does the alkyl chain length on the azobenzene unit of these di-mesogenic compounds affect their liquid crystal properties?
A: Research indicates that the presence and length of the alkyl chain on the azobenzene unit significantly influence the mesophase behavior [, ]. Compounds lacking an alkyl chain on the azobenzene unit exhibit both cholesteric and smectic phases. In contrast, those with an alkyl chain ranging from butyl to hexadecyl only display a smectic phase [, ]. This suggests that the alkyl chain length plays a crucial role in dictating the self-assembly and resulting mesophase structures formed by these compounds.
Q3: Can the cholesteric properties of these di-mesogenic compounds be controlled by external stimuli?
A: Yes, the cholesteric properties of these compounds can be controlled photochemically. When exposed to UV light (366 nm), the azobenzene unit within the di-mesogenic compound undergoes isomerization from the trans to the cis form [, ]. This isomerization induces a shift in the cholesteric reflection band towards shorter wavelengths, effectively changing the perceived color of the material [, ]. This photo-responsive behavior has potential applications in rewritable color display technologies.
Q4: What is the significance of incorporating these di-mesogenic compounds into a host cholesteric liquid crystal?
A: Incorporating small amounts (2-10 wt%) of these di-mesogenic compounds into a host cholesteric liquid crystal, like a dicholesteryl ester, imparts photo-responsiveness to the resulting mixture []. This means that the mixture's cholesteric properties, like its helical pitch and reflected color, become sensitive to UV light exposure. Furthermore, the mixture can be rapidly cooled from its liquid crystalline state to a glassy state while retaining the photo-induced changes in cholesteric properties []. This stability in the glassy state makes these mixtures particularly attractive for applications like full-color rewritable recording materials.
Q5: Are there any historical milestones or cross-disciplinary applications related to this compound or its derivatives?
A: While the provided research focuses on the use of this compound derivatives in liquid crystal applications, a separate study investigated the metabolic breakdown of diynes, including this compound, in rats []. This highlights the compound's relevance in understanding biological processes and potential applications beyond material science. The diverse applications of this compound and its derivatives emphasize the importance of cross-disciplinary research and collaboration in advancing our understanding and utilization of such compounds.
Disclaimer and Information on In-Vitro Research Products
Please be aware that all articles and product information presented on BenchChem are intended solely for informational purposes. The products available for purchase on BenchChem are specifically designed for in-vitro studies, which are conducted outside of living organisms. In-vitro studies, derived from the Latin term "in glass," involve experiments performed in controlled laboratory settings using cells or tissues. It is important to note that these products are not categorized as medicines or drugs, and they have not received approval from the FDA for the prevention, treatment, or cure of any medical condition, ailment, or disease. We must emphasize that any form of bodily introduction of these products into humans or animals is strictly prohibited by law. It is essential to adhere to these guidelines to ensure compliance with legal and ethical standards in research and experimentation.