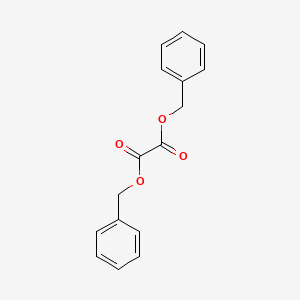
Dibenzyl oxalate
Overview
Description
Dibenzyl oxalate is a chemical compound with the molecular formula C16H14O4 . It has an average mass of 270.280 Da and a monoisotopic mass of 270.089203 Da . It is also known by other names such as dibenzyl ethanedioate and ethanedioic acid, bis(phenylmethyl) ester .
Synthesis Analysis
The synthesis of Dibenzyl oxalate involves a novel, fast, and simple esterification procedure . The esterification comprises in situ generation of HCl, which catalyzes the Fischer esterification with benzyl alcohol . The yield of the synthesized compound was determined to be 60 ± 5% via NMR spectroscopy .
Molecular Structure Analysis
The molecular structure of Dibenzyl oxalate is represented by the linear formula (CO2CH2C6H5)2 . It has a molecular weight of 270.28 .
Physical And Chemical Properties Analysis
Dibenzyl oxalate is a white flaked crystalline solid . It has a melting point of 80-82 °C (lit.) and a boiling point of 235 °C/14 mmHg (lit.) . It has a density of 1.212 and a refractive index of 1.5447 (estimate) .
Scientific Research Applications
Synthesis Methods
Dibenzyl oxalate can be synthesized from oxalic acid and benzyl alcohol using various catalysts. The optimal reaction conditions involve a mole ratio of oxalic acid to benzyl alcohol of 1:2.2, using p-toluene sulphonic acid as a catalyst and benzene as a water-carrying agent, leading to a high yield of 96.2% (Yan Tao, 2009). Additionally, dibenzyls, essential building blocks in organic synthesis, can be prepared via nickel-catalyzed and dimethyl oxalate-assisted dynamic kinetic homocoupling of benzyl alcohols (Xing‐Zhong Shu et al., 2021).
Environmental and Analytical Applications
Oxalate, a component of dibenzyl oxalate, is significant in environmental and analytical applications. A "lab in a tube" platform using a stimuli-responsive hydrogel-based kit was developed for on-site monitoring of oxalate, crucial for accurately screening trace residues that may cause renal injury (Rui Jin et al., 2020). Furthermore, an oxalate-based deep eutectic solvent exhibited high activity in the oxidative desulfurization of dibenzothiophene, providing a promising and environmentally friendly approach for fuel desulfurization (H. Lü et al., 2015).
Biomedical Applications
In biomedical research, oxalate, which is related to dibenzyl oxalate, plays a role in various processes. Oxalate oxidase from barley roots was studied for its molecular, catalytic, and binding properties, contributing to the understanding of enzyme functionality in biological systems (V. P. Kotsira & Y. Clonis, 1997). Additionally, palladium(II) oxalato complexes involving adenine-based ligands showed significant cytotoxicity against human chronic myelogenous leukaemia and breast adenocarcinoma cancer cell lines, indicating potential applications in cancer treatment (P. Štarha et al., 2009).
Safety and Hazards
Dibenzyl oxalate is considered hazardous according to the 2012 OSHA Hazard Communication Standard (29 CFR 1910.1200) . It can cause skin irritation, serious eye irritation, and may cause respiratory irritation . It is recommended to wear personal protective equipment, ensure adequate ventilation, avoid dust formation, and avoid contact with skin, eyes, or clothing .
properties
IUPAC Name |
dibenzyl oxalate | |
---|---|---|
Source | PubChem | |
URL | https://pubchem.ncbi.nlm.nih.gov | |
Description | Data deposited in or computed by PubChem | |
InChI |
InChI=1S/C16H14O4/c17-15(19-11-13-7-3-1-4-8-13)16(18)20-12-14-9-5-2-6-10-14/h1-10H,11-12H2 | |
Source | PubChem | |
URL | https://pubchem.ncbi.nlm.nih.gov | |
Description | Data deposited in or computed by PubChem | |
InChI Key |
ZYZXGWGQYNTGAU-UHFFFAOYSA-N | |
Source | PubChem | |
URL | https://pubchem.ncbi.nlm.nih.gov | |
Description | Data deposited in or computed by PubChem | |
Canonical SMILES |
C1=CC=C(C=C1)COC(=O)C(=O)OCC2=CC=CC=C2 | |
Source | PubChem | |
URL | https://pubchem.ncbi.nlm.nih.gov | |
Description | Data deposited in or computed by PubChem | |
Molecular Formula |
C16H14O4 | |
Source | PubChem | |
URL | https://pubchem.ncbi.nlm.nih.gov | |
Description | Data deposited in or computed by PubChem | |
DSSTOX Substance ID |
DTXSID4038926 | |
Record name | Dibenzyl oxalate | |
Source | EPA DSSTox | |
URL | https://comptox.epa.gov/dashboard/DTXSID4038926 | |
Description | DSSTox provides a high quality public chemistry resource for supporting improved predictive toxicology. | |
Molecular Weight |
270.28 g/mol | |
Source | PubChem | |
URL | https://pubchem.ncbi.nlm.nih.gov | |
Description | Data deposited in or computed by PubChem | |
Product Name |
Dibenzyl oxalate | |
CAS RN |
7579-36-4 | |
Record name | Dibenzyl oxalate | |
Source | CAS Common Chemistry | |
URL | https://commonchemistry.cas.org/detail?cas_rn=7579-36-4 | |
Description | CAS Common Chemistry is an open community resource for accessing chemical information. Nearly 500,000 chemical substances from CAS REGISTRY cover areas of community interest, including common and frequently regulated chemicals, and those relevant to high school and undergraduate chemistry classes. This chemical information, curated by our expert scientists, is provided in alignment with our mission as a division of the American Chemical Society. | |
Explanation | The data from CAS Common Chemistry is provided under a CC-BY-NC 4.0 license, unless otherwise stated. | |
Record name | Dibenzyl oxalate | |
Source | ChemIDplus | |
URL | https://pubchem.ncbi.nlm.nih.gov/substance/?source=chemidplus&sourceid=0007579364 | |
Description | ChemIDplus is a free, web search system that provides access to the structure and nomenclature authority files used for the identification of chemical substances cited in National Library of Medicine (NLM) databases, including the TOXNET system. | |
Record name | Dibenzyl oxalate | |
Source | DTP/NCI | |
URL | https://dtp.cancer.gov/dtpstandard/servlet/dwindex?searchtype=NSC&outputformat=html&searchlist=52550 | |
Description | The NCI Development Therapeutics Program (DTP) provides services and resources to the academic and private-sector research communities worldwide to facilitate the discovery and development of new cancer therapeutic agents. | |
Explanation | Unless otherwise indicated, all text within NCI products is free of copyright and may be reused without our permission. Credit the National Cancer Institute as the source. | |
Record name | Ethanedioic acid, 1,2-bis(phenylmethyl) ester | |
Source | EPA Chemicals under the TSCA | |
URL | https://www.epa.gov/chemicals-under-tsca | |
Description | EPA Chemicals under the Toxic Substances Control Act (TSCA) collection contains information on chemicals and their regulations under TSCA, including non-confidential content from the TSCA Chemical Substance Inventory and Chemical Data Reporting. | |
Record name | Dibenzyl oxalate | |
Source | EPA DSSTox | |
URL | https://comptox.epa.gov/dashboard/DTXSID4038926 | |
Description | DSSTox provides a high quality public chemistry resource for supporting improved predictive toxicology. | |
Record name | Ethanedioic acid, 1,2-bis(phenylmethyl) ester | |
Source | European Chemicals Agency (ECHA) | |
URL | https://echa.europa.eu/information-on-chemicals | |
Description | The European Chemicals Agency (ECHA) is an agency of the European Union which is the driving force among regulatory authorities in implementing the EU's groundbreaking chemicals legislation for the benefit of human health and the environment as well as for innovation and competitiveness. | |
Explanation | Use of the information, documents and data from the ECHA website is subject to the terms and conditions of this Legal Notice, and subject to other binding limitations provided for under applicable law, the information, documents and data made available on the ECHA website may be reproduced, distributed and/or used, totally or in part, for non-commercial purposes provided that ECHA is acknowledged as the source: "Source: European Chemicals Agency, http://echa.europa.eu/". Such acknowledgement must be included in each copy of the material. ECHA permits and encourages organisations and individuals to create links to the ECHA website under the following cumulative conditions: Links can only be made to webpages that provide a link to the Legal Notice page. | |
Record name | DIBENZYL OXALATE | |
Source | FDA Global Substance Registration System (GSRS) | |
URL | https://gsrs.ncats.nih.gov/ginas/app/beta/substances/9A31Q81DDC | |
Description | The FDA Global Substance Registration System (GSRS) enables the efficient and accurate exchange of information on what substances are in regulated products. Instead of relying on names, which vary across regulatory domains, countries, and regions, the GSRS knowledge base makes it possible for substances to be defined by standardized, scientific descriptions. | |
Explanation | Unless otherwise noted, the contents of the FDA website (www.fda.gov), both text and graphics, are not copyrighted. They are in the public domain and may be republished, reprinted and otherwise used freely by anyone without the need to obtain permission from FDA. Credit to the U.S. Food and Drug Administration as the source is appreciated but not required. | |
Retrosynthesis Analysis
AI-Powered Synthesis Planning: Our tool employs the Template_relevance Pistachio, Template_relevance Bkms_metabolic, Template_relevance Pistachio_ringbreaker, Template_relevance Reaxys, Template_relevance Reaxys_biocatalysis model, leveraging a vast database of chemical reactions to predict feasible synthetic routes.
One-Step Synthesis Focus: Specifically designed for one-step synthesis, it provides concise and direct routes for your target compounds, streamlining the synthesis process.
Accurate Predictions: Utilizing the extensive PISTACHIO, BKMS_METABOLIC, PISTACHIO_RINGBREAKER, REAXYS, REAXYS_BIOCATALYSIS database, our tool offers high-accuracy predictions, reflecting the latest in chemical research and data.
Strategy Settings
Precursor scoring | Relevance Heuristic |
---|---|
Min. plausibility | 0.01 |
Model | Template_relevance |
Template Set | Pistachio/Bkms_metabolic/Pistachio_ringbreaker/Reaxys/Reaxys_biocatalysis |
Top-N result to add to graph | 6 |
Feasible Synthetic Routes
Q & A
Q1: What are the optimal conditions for synthesizing dibenzyl oxalate?
A1: Research has shown that dibenzyl oxalate can be effectively synthesized using oxalic acid and benzyl alcohol as starting materials []. The optimal conditions involve a molar ratio of 1:2.2 (oxalic acid: benzyl alcohol), 0.15g of p-toluene sulphonic acid as a catalyst, and benzene (40 mL) as the water-carrying agent. Under these conditions, a reaction time of 40 minutes can yield up to 96.2% dibenzyl oxalate [].
Q2: How does dibenzyl oxalate decompose in the presence of iodine?
A2: Interestingly, dibenzyl oxalate, stable at certain temperatures, undergoes decomposition when iodine is introduced []. Unlike some other dialkyl oxalates that yield corresponding iodides, dibenzyl oxalate forms diphenylmethane in this reaction. The mechanism behind this iodine-induced decomposition is a subject of ongoing scientific investigation [].
Q3: Can dibenzyl oxalate be used in drug delivery systems?
A3: While the provided abstracts don't directly address this, one study highlights the use of dibenzyl oxalate in a biomimetic nanosystem for potential pneumonia treatment []. In this system, dibenzyl oxalate is incorporated into a nucleus-targeted nanosystem designed to deliver hypericin in response to adenosine triphosphate (ATP) and reactive oxygen species []. This suggests potential applications of dibenzyl oxalate in targeted drug delivery, particularly for intracellular delivery.
Q4: What analytical techniques are used to characterize dibenzyl oxalate?
A4: Several methods have been employed to characterize dibenzyl oxalate. Melting point measurement confirms its identity []. Additionally, spectroscopic techniques such as Infrared spectroscopy (IR) and Liquid Chromatography-Mass Spectrometry (LC-MS) are used to determine the structure of the synthesized product []. These techniques provide valuable insights into the compound's properties and purity.
Disclaimer and Information on In-Vitro Research Products
Please be aware that all articles and product information presented on BenchChem are intended solely for informational purposes. The products available for purchase on BenchChem are specifically designed for in-vitro studies, which are conducted outside of living organisms. In-vitro studies, derived from the Latin term "in glass," involve experiments performed in controlled laboratory settings using cells or tissues. It is important to note that these products are not categorized as medicines or drugs, and they have not received approval from the FDA for the prevention, treatment, or cure of any medical condition, ailment, or disease. We must emphasize that any form of bodily introduction of these products into humans or animals is strictly prohibited by law. It is essential to adhere to these guidelines to ensure compliance with legal and ethical standards in research and experimentation.