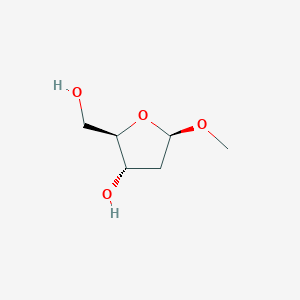
Methyl-2-deoxy-beta-D-ribofuranoside
Overview
Description
Methyl-2-deoxy-beta-D-ribofuranoside is a chemical compound with the molecular formula C6H12O4 and a molecular weight of 148.1571 g/mol . It is a derivative of ribose, a sugar that is a fundamental component of RNA. This compound is characterized by the absence of a hydroxyl group at the second carbon position, which distinguishes it from its parent sugar, ribose .
Mechanism of Action
Target of Action
Methyl-2-deoxy-beta-D-ribofuranoside is a type of purine nucleoside analog . These analogs have a broad spectrum of anti-tumor activity, targeting indolent lymphatic system malignant tumors . The anti-cancer mechanism in this process relies on inhibiting DNA synthesis and inducing apoptosis .
Mode of Action
The mode of action of this compound involves its interaction with the DNA synthesis process. By inhibiting DNA synthesis, it disrupts the normal cell cycle, leading to cell death or apoptosis . This is particularly effective against cancer cells, which have a high rate of DNA synthesis.
Biochemical Pathways
This compound affects the DNA synthesis pathway. By inhibiting this pathway, it disrupts the normal functioning of cells, leading to apoptosis . The downstream effects include the death of cancer cells and a reduction in tumor size.
Result of Action
The result of this compound’s action is the induction of apoptosis in cancer cells . By inhibiting DNA synthesis, it disrupts the normal cell cycle, leading to cell death. This results in a reduction in tumor size and potentially the elimination of the cancer.
Preparation Methods
Synthetic Routes and Reaction Conditions
The synthesis of Methyl-2-deoxy-beta-D-ribofuranoside typically involves the methylation of 2-deoxy-D-ribose. One common method is the reaction of 2-deoxy-D-ribose with methanol in the presence of an acid catalyst, such as hydrochloric acid, to form the methyl glycoside . The reaction conditions often include refluxing the mixture for several hours to ensure complete conversion.
Industrial Production Methods
Industrial production of this compound follows similar synthetic routes but on a larger scale. The process involves the use of industrial-grade reagents and optimized reaction conditions to maximize yield and purity. The product is then purified through crystallization or chromatography techniques .
Chemical Reactions Analysis
Types of Reactions
Methyl-2-deoxy-beta-D-ribofuranoside undergoes various chemical reactions, including:
Oxidation: The compound can be oxidized to form corresponding carboxylic acids.
Reduction: Reduction reactions can convert it into alcohols.
Substitution: It can undergo nucleophilic substitution reactions, where the methoxy group can be replaced by other nucleophiles.
Common Reagents and Conditions
Oxidation: Common oxidizing agents include potassium permanganate and chromium trioxide.
Reduction: Reducing agents such as sodium borohydride or lithium aluminum hydride are typically used.
Substitution: Nucleophiles like halides, amines, or thiols can be used under basic or acidic conditions.
Major Products Formed
The major products formed from these reactions depend on the specific reagents and conditions used. For example, oxidation can yield carboxylic acids, while reduction can produce alcohols .
Scientific Research Applications
Methyl-2-deoxy-beta-D-ribofuranoside has several applications in scientific research:
Chemistry: It is used as a building block in the synthesis of more complex molecules.
Biology: The compound is studied for its role in biochemical pathways and its interactions with enzymes.
Medicine: It is investigated for its potential therapeutic applications, including as a precursor for antiviral and anticancer agents.
Comparison with Similar Compounds
Similar Compounds
Methyl-beta-D-ribofuranoside: Similar in structure but with a hydroxyl group at the second carbon position.
2-Deoxy-D-ribose: Lacks the methoxy group but shares the deoxy structure at the second carbon.
Methyl-2,3-O-isopropylidene-beta-D-ribofuranoside: A derivative with additional protective groups.
Uniqueness
Methyl-2-deoxy-beta-D-ribofuranoside is unique due to its specific structural features, which confer distinct chemical and biological properties. Its deoxy nature at the second carbon and the presence of a methoxy group make it a valuable compound for various synthetic and research applications .
Properties
IUPAC Name |
2-(hydroxymethyl)-5-methoxyoxolan-3-ol | |
---|---|---|
Details | Computed by LexiChem 2.6.6 (PubChem release 2019.06.18) | |
Source | PubChem | |
URL | https://pubchem.ncbi.nlm.nih.gov | |
Description | Data deposited in or computed by PubChem | |
InChI |
InChI=1S/C6H12O4/c1-9-6-2-4(8)5(3-7)10-6/h4-8H,2-3H2,1H3 | |
Details | Computed by InChI 1.0.5 (PubChem release 2019.06.18) | |
Source | PubChem | |
URL | https://pubchem.ncbi.nlm.nih.gov | |
Description | Data deposited in or computed by PubChem | |
InChI Key |
NVGJZDFWPSOTHM-UHFFFAOYSA-N | |
Details | Computed by InChI 1.0.5 (PubChem release 2019.06.18) | |
Source | PubChem | |
URL | https://pubchem.ncbi.nlm.nih.gov | |
Description | Data deposited in or computed by PubChem | |
Canonical SMILES |
COC1CC(C(O1)CO)O | |
Details | Computed by OEChem 2.1.5 (PubChem release 2019.06.18) | |
Source | PubChem | |
URL | https://pubchem.ncbi.nlm.nih.gov | |
Description | Data deposited in or computed by PubChem | |
Molecular Formula |
C6H12O4 | |
Details | Computed by PubChem 2.1 (PubChem release 2019.06.18) | |
Source | PubChem | |
URL | https://pubchem.ncbi.nlm.nih.gov | |
Description | Data deposited in or computed by PubChem | |
Molecular Weight |
148.16 g/mol | |
Details | Computed by PubChem 2.1 (PubChem release 2021.05.07) | |
Source | PubChem | |
URL | https://pubchem.ncbi.nlm.nih.gov | |
Description | Data deposited in or computed by PubChem | |
CAS No. |
51255-18-6 | |
Record name | NSC68139 | |
Source | DTP/NCI | |
URL | https://dtp.cancer.gov/dtpstandard/servlet/dwindex?searchtype=NSC&outputformat=html&searchlist=68139 | |
Description | The NCI Development Therapeutics Program (DTP) provides services and resources to the academic and private-sector research communities worldwide to facilitate the discovery and development of new cancer therapeutic agents. | |
Explanation | Unless otherwise indicated, all text within NCI products is free of copyright and may be reused without our permission. Credit the National Cancer Institute as the source. | |
Retrosynthesis Analysis
AI-Powered Synthesis Planning: Our tool employs the Template_relevance Pistachio, Template_relevance Bkms_metabolic, Template_relevance Pistachio_ringbreaker, Template_relevance Reaxys, Template_relevance Reaxys_biocatalysis model, leveraging a vast database of chemical reactions to predict feasible synthetic routes.
One-Step Synthesis Focus: Specifically designed for one-step synthesis, it provides concise and direct routes for your target compounds, streamlining the synthesis process.
Accurate Predictions: Utilizing the extensive PISTACHIO, BKMS_METABOLIC, PISTACHIO_RINGBREAKER, REAXYS, REAXYS_BIOCATALYSIS database, our tool offers high-accuracy predictions, reflecting the latest in chemical research and data.
Strategy Settings
Precursor scoring | Relevance Heuristic |
---|---|
Min. plausibility | 0.01 |
Model | Template_relevance |
Template Set | Pistachio/Bkms_metabolic/Pistachio_ringbreaker/Reaxys/Reaxys_biocatalysis |
Top-N result to add to graph | 6 |
Feasible Synthetic Routes
Q1: What is the structural characterization of methyl 2'-deoxy-β-D-ribofuranoside?
A1: While the article doesn't explicitly provide the molecular formula and weight, it focuses on the conformational analysis of methyl 2'-deoxy-β-D-ribofuranoside using proton magnetic resonance spectroscopy. [] This technique provides valuable information about the spatial arrangement of atoms within the molecule. The study utilizes this spectroscopic data alongside molecular mechanics calculations to determine the preferred conformations of the molecule in solution.
Q2: How does the study utilize computational chemistry to understand methyl 2'-deoxy-β-D-ribofuranoside?
A2: The research employs molecular mechanics calculations to complement the proton magnetic resonance spectroscopy data. [] This computational approach helps researchers explore different possible conformations of methyl 2'-deoxy-β-D-ribofuranoside and calculate their relative energies. By comparing these calculated energies with experimental data obtained from spectroscopy, the study identifies the most stable and likely conformations adopted by the molecule. This information is crucial for understanding the molecule's behavior in various chemical and biological contexts.
Disclaimer and Information on In-Vitro Research Products
Please be aware that all articles and product information presented on BenchChem are intended solely for informational purposes. The products available for purchase on BenchChem are specifically designed for in-vitro studies, which are conducted outside of living organisms. In-vitro studies, derived from the Latin term "in glass," involve experiments performed in controlled laboratory settings using cells or tissues. It is important to note that these products are not categorized as medicines or drugs, and they have not received approval from the FDA for the prevention, treatment, or cure of any medical condition, ailment, or disease. We must emphasize that any form of bodily introduction of these products into humans or animals is strictly prohibited by law. It is essential to adhere to these guidelines to ensure compliance with legal and ethical standards in research and experimentation.