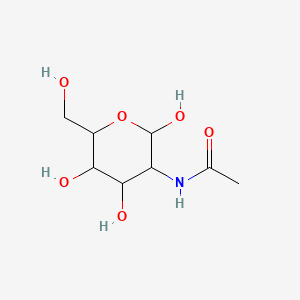
N-Acetyl-D-Galactosamine
Overview
Description
N-Acetyl-D-Galactosamine (GalNAc) is a monosaccharide derivative of galactose, characterized by an N-acetyl group (-NHCOCH₃) at the C2 position. Its molecular formula is C₈H₁₅NO₆, with a molecular weight of 221.21 g/mol (CAS: 14215-68-0) . GalNAc is a critical component of glycoproteins and proteoglycans, serving as the initial sugar in mucin-type O-linked glycosylation, where it is transferred to serine or threonine residues by UDP-GalNAc:polypeptide N-acetylgalactosaminyltransferases (GalNAc-Ts) .
Preparation Methods
Enzymatic Synthesis of UDP-N-Acetyl-D-Galactosamine
One prominent method involves enzymatic preparation of UDP-N-Acetyl-D-Galactosamine, a nucleotide sugar derivative critical in glycosylation processes.
- Process Overview : UDP-N-Acetyl-D-Galactosamine is synthesized by acetylation of UDP-galactosamine using radiolabeled [1-14C]acetate and the coupling agent N-ethoxycarbonyl-2-ethoxy-1,2-dihydroquinoline.
- Precursor Preparation : UDP-galactosamine itself is enzymatically prepared using galactokinase and galactose-1-phosphate uridyltransferase.
- Yield and Purity : The overall yield based on [1-14C]acetate exceeds 80%, with products characterized by ion-exchange and paper chromatography confirming high substrate activity in glycosyl transferase systems.
- Significance : This method allows for high-specific-activity labeled compounds useful in biochemical assays and metabolic studies.
Chemical Synthesis via Acetylation of D-Galactosamine Hydrochloride
A widely applied industrial and laboratory method involves direct acetylation of D-galactosamine hydrochloride to obtain N-Acetyl-D-Galactosamine pentaacetate, followed by deacetylation.
General Procedure
- Reactants : D-galactosamine hydrochloride, acetic anhydride (acetylating agent), pyridine (solvent and base), and 4-dimethylaminopyridine (catalyst).
- Reaction Conditions : Typically performed at low temperature (0°C) initially, followed by stirring at room temperature for 10–12 hours.
- Post-Reaction Processing : Solvent removal under reduced pressure, washing with toluene, and treatment with anhydrous methanol to precipitate the acetylated product.
- Deacetylation : The pentaacetate intermediate is then subjected to methanolysis using sodium methoxide in methanol to yield this compound.
Representative Data from Industrial-Scale Examples
Step | Conditions/Materials | Yield/Content |
---|---|---|
Acetylation (S1) | 10 kg D-galactosamine hydrochloride, 30 kg acetic anhydride, 30 kg pyridine, 200 g 4-dimethylaminopyridine, 0–25°C, 10–12 h | White solid pentaacetate, 97.8–98.2% purity, yield ~93.6% |
Deacetylation (S2) | 10 kg pentaacetate, 70 kg methanol, 3.5 kg sodium methoxide, room temperature | This compound with high purity |
- Additives : Tris(2-chloroethyl) phosphate added in some protocols (0.01–0.02 eq) improves yield and purity.
- Advantages : Mild conditions, scalable production, and efficient separation of overacetylated byproducts.
- Challenges Addressed : This method solves issues related to long reaction times, difficult separation of peracetylated products, and yield reduction due to overacetylation.
Fluorinated Analog Synthesis for Structural Modifications
Recent research has explored the synthesis of fluorinated this compound analogs to modulate biological properties such as protein affinity and metabolic stability.
- Synthetic Route : Starting from deoxyfluorinated 1,6-anhydro-2-azido-β-D-hexopyranose precursors, the key intermediates are fluorinated glucosazide and galactosazide thioglycosides.
- Key Reactions : Nucleophilic deoxyfluorination using reagents like DAST (diethylaminosulfur trifluoride), followed by thioglycoside hydrolysis and azide-to-acetamide transformation.
- Outcome : A series of mono-, di-, and trifluorinated this compound analogs with potential for enhanced biochemical applications.
Comparative Summary of Preparation Methods
Method | Key Features | Advantages | Limitations |
---|---|---|---|
Enzymatic synthesis | Uses galactokinase, uridyltransferase enzymes; acetylation with labeled acetate | High specificity; useful for labeled compounds | Requires enzymes; complex setup |
Chemical acetylation of D-galactosamine hydrochloride | Acetic anhydride/pyridine acetylation; methanolysis deacetylation | Scalable; mild conditions; high purity and yield | Requires careful control to avoid overacetylation |
Fluorinated analog synthesis | Multi-step fluorination and azide transformations | Enables structural modification; improved properties | Complex synthesis; specialized reagents |
Detailed Research Findings and Notes
- The enzymatic method achieves >80% yield with high specific activity, critical for tracer studies in glycobiology.
- The chemical acetylation method achieves >93% yield of pentaacetate intermediate with purity >97%, enabling efficient production of this compound at industrial scale.
- Addition of catalysts such as 4-dimethylaminopyridine and tris(2-chloroethyl) phosphate improves reaction efficiency and product purity.
- Fluorinated analog synthesis expands the chemical space of this compound derivatives, allowing for enhanced biological studies and potential therapeutic applications.
Chemical Reactions Analysis
Types of Reactions: N-Acetyl-D-Galactosamine undergoes various chemical reactions, including oxidation, reduction, and substitution. It is also involved in glycosylation reactions, where it serves as a donor molecule in the formation of glycosidic bonds.
Common Reagents and Conditions: Common reagents used in the reactions involving this compound include acetic anhydride, 4-dimethylaminopyridine, and rare earth metal triflates. The conditions for these reactions typically involve mild temperatures and specific pH levels to ensure high yield and specificity .
Major Products: The major products formed from the reactions involving this compound include various glycosides and glycosylated proteins. These products are essential in numerous biological processes and have significant applications in medicine and biotechnology .
Scientific Research Applications
Therapeutic Applications
1.1 Oligonucleotide Delivery Systems
N-Acetyl-D-galactosamine has emerged as a key component in the development of targeted oligonucleotide therapeutics. It enhances the delivery of antisense oligonucleotides (ASOs) and small interfering RNAs (siRNAs) to hepatic targets, significantly improving their pharmacokinetics and efficacy. For instance, several GalNAc-conjugated therapeutics have progressed to clinical trials for conditions such as hyperlipidemia and viral infections. The technology allows for a reduction in dosing frequency, with some therapies requiring only two doses per year .
1.2 Cancer Treatment
Research indicates that GalNAc can be utilized in anticancer therapies due to its ability to target specific cancer cell types through glycan recognition. For example, certain plant-derived compounds that include GalNAc are being investigated for their potential to inhibit tumor growth by modulating immune responses and inducing apoptosis in cancer cells . The incorporation of GalNAc into therapeutic agents enhances their specificity and reduces off-target effects.
Biochemical Research
2.1 Enzyme Characterization
GalNAc is involved in various enzymatic processes, including those mediated by N-acetyl-D-galactosaminyltransferases, which play a role in blood group antigen expression. Studies have shown that these enzymes catalyze the transfer of GalNAc to mucin receptors, influencing blood group A antigenicity . Understanding these enzymatic pathways is crucial for developing blood type-specific therapies and diagnostics.
2.2 Bacterial Metabolism
The utilization of this compound by bacteria like Escherichia coli has been extensively studied, revealing insights into carbohydrate metabolism and gene regulation. The identification of specific gene clusters responsible for GalNAc catabolism has implications for understanding microbial ecology and developing biotechnological applications involving microbial fermentation processes .
Structural Biology
3.1 Glycosylation Studies
GalNAc is a fundamental component of O-glycosylation processes in proteins. Its incorporation into peptides influences protein folding and function. Studies have demonstrated that specific GalNAc transferases mediate this process, impacting various biological functions including cell signaling and immune responses .
Data Tables
The following table summarizes key findings related to the applications of this compound:
Mechanism of Action
N-Acetyl-D-Galactosamine exerts its effects by binding to specific receptors on the surface of cells, such as the asialoglycoprotein receptors on hepatocytes. This binding facilitates the internalization of the compound and its associated molecules, leading to various cellular responses. The molecular targets and pathways involved include the regulation of glycosylation processes and the modulation of immune responses .
Comparison with Similar Compounds
Comparison with Structurally Similar Compounds
N-Acetyl-D-Glucosamine (GlcNAc)
Structural Differences :
- GalNAc and GlcNAc are epimers , differing at the C4 hydroxyl configuration: axial in GlcNAc (glucose-derived) vs. equatorial in GalNAc (galactose-derived) .
Property | GalNAc | GlcNAc |
---|---|---|
Epimeric Position | C4 (equatorial OH) | C4 (axial OH) |
Key Biological Role | O-glycosylation initiation | Hyaluronan, N-glycan branching |
Lectin Specificity | ASGPRs, ML-II/III lectins | Wheat germ agglutinin (WGA) |
Functional Contrasts :
- Glycosaminoglycans (GAGs): GalNAc is sulfated in chondroitin sulfate (GlcA-GalNAc disaccharides), while GlcNAc is a component of hyaluronan (GlcA-GlcNAc) .
- Enzymatic Specificity: GalNAc-T3 preferentially glycosylates peptide motifs in secretory adenocarcinomas, unlike GlcNAc-transferases, which mediate N-glycan processing .
D-Galactosamine
Structural Differences :
- D-Galactosamine lacks the N-acetyl group at C2, possessing a free amino group (-NH₂) instead .
Property | GalNAc | D-Galactosamine |
---|---|---|
C2 Functional Group | N-acetyl (-NHCOCH₃) | Amino (-NH₂) |
Pharmacological Use | Tumor targeting, glycobiology | Liver injury models (e.g., D-galactosamine-induced hepatitis) |
Metabolic and Functional Insights :
- Lectin Interactions : The Entamoeba histolytica lectin binds both compounds, but GalNAc is a stronger inhibitor due to its acetyl group enhancing affinity .
D-Galactose
Structural Differences :
Property | GalNAc | D-Galactose |
---|---|---|
C2 Functional Group | N-acetyl (-NHCOCH₃) | Hydroxyl (-OH) |
Lectin Binding | High-affinity ASGPR ligand | Recognized by galectins |
Sulfated Derivatives in Chondroitin Sulfate
Sulfation Patterns :
- GalNAc in chondroitin sulfate is sulfated at C4 or C6 , influencing charge density and extracellular matrix interactions .
Sulfation Type | Biological Impact |
---|---|
4-O-sulfation (Chondroitin-4-sulfate) | Enhances collagen binding and tissue repair |
6-O-sulfation (Chondroitin-6-sulfate) | Modulates neuronal growth and immune responses |
Comparison with Non-Sulfated GalNAc:
- Sulfation alters solubility and protein-binding capacity, enabling specialized roles in cartilage (chondroitin sulfate) vs. cancer (unsulfated GalNAc glycans) .
Table 2: Enzymatic and Receptor Interactions
Biological Activity
N-Acetyl-D-galactosamine (GalNAc) is a monosaccharide that plays a significant role in various biological processes. This article delves into its biological activities, mechanisms of action, and applications in therapeutics, supported by case studies and research findings.
Overview of this compound
GalNAc is an amino sugar derived from galactose, widely recognized for its involvement in glycosylation processes. It serves as a substrate for glycosyltransferases, which are enzymes that facilitate the addition of carbohydrate moieties to proteins and lipids, thereby influencing cellular interactions and functions.
Biological Activities
1. Glycosylation and Cellular Functions
GalNAc is essential for the synthesis of glycoproteins and glycolipids. It is particularly crucial in the formation of mucins, which are glycoproteins that play protective roles in epithelial tissues. The human genome encodes multiple GalNAc transferases (GalNAc-Ts), which exhibit distinct substrate specificities and expression patterns. For instance, GalNAc-T16 is abundantly expressed in the heart and has robust transferase activity, while GalNAc-T18 shows weak activity with specific peptides .
2. Immunomodulatory Effects
Research indicates that GalNAc can modulate immune responses. It has been shown to inhibit the agglutination of leukocytes and erythrocytes by phytohemagglutinin, suggesting a potential role in regulating immune cell interactions . This property may have implications for therapeutic strategies aimed at controlling inflammatory responses.
3. Anticoagulant Properties
GalNAc exhibits anticoagulant activity, which has been attributed to its structural properties that influence blood coagulation pathways. Studies have demonstrated its ability to interact with various components of the coagulation cascade, thereby providing a basis for its use in developing anticoagulant therapies .
The biological activity of GalNAc is mediated through several mechanisms:
- Binding to Receptors: GalNAc specifically binds to receptors on hepatocytes, facilitating targeted delivery of therapeutic agents. For example, in RNA interference (RNAi) therapies, GalNAc-conjugated molecules can effectively silence target genes within liver cells .
- Enzymatic Activity: The enzymatic activities associated with GalNAc transferases are crucial for glycan formation on proteins. These activities are regulated by substrate availability and cellular conditions, leading to diverse biological outcomes .
Case Study 1: RNAi Therapeutics
Dicerna Pharmaceuticals has developed a platform utilizing GalNAc for targeted gene silencing in liver cells. Their GalXC RNAi molecules demonstrate high potency and specificity due to the incorporation of GalNAc, achieving effective gene silencing with minimal dosing frequency . In vivo studies have shown EC50 values typically ranging from 0.1 to 1.0 mg/kg body weight.
Case Study 2: Glycosylation Disorders
GalNAc's role in glycosylation has been explored in various disorders. Abnormalities in GalNAc transferase activity can lead to diseases such as congenital disorders of glycosylation (CDG). Research highlights the importance of understanding these pathways for developing targeted therapies .
Data Table: Biological Activities of this compound
Q & A
Q. Basic: What are the primary biochemical roles of GalNAc in glycoprotein synthesis, and how can its incorporation be experimentally validated?
GalNAc serves as a critical substrate for O-linked glycosylation, where it is transferred to serine/threonine residues of proteins via GalNAc-transferases (GalNAc-Ts) . To validate its incorporation, researchers employ:
- Lectin-affinity chromatography : Use GalNAc-specific lectins (e.g., Dolichos biflorus agglutinin) in pull-down assays to isolate glycoproteins .
- Mass spectrometry : Analyze tryptic digests of glycoproteins to identify GalNAc-modified peptides via collision-induced dissociation (CID) or electron-transfer dissociation (ETD) .
- Enzymatic inhibition : Treat cells with GalNAc analogs (e.g., benzyl-α-GalNAc) to competitively inhibit glycosylation, followed by Western blotting to detect reduced glycan signals .
Q. Advanced: How can CRISPRa systems be optimized to study GalNAc-T isoform-specific functions in cellular models?
CRISPR activation (CRISPRa) systems targeting GALNT genes require:
- sgRNA design : Prioritize regions upstream of the transcription start site (TSS) or within enhancer regions of GALNT5 or GALNT17 to maximize activation efficiency .
- Delivery optimization : Use lentiviral vectors with dCas9-VP64 or SAM complex (dCas9-MS2-p65-HSF1) for stable overexpression in cell lines like HEK293 or HepG2 .
- Functional validation : Quantify GalNAc-T activity via UDP-GalNAc consumption assays or monitor O-glycan profiles using HPLC or lectin microarrays .
Q. Basic: What methodologies are recommended for detecting and quantifying free GalNAc in biological fluids?
- HPLC with PMP derivatization : Derivatize GalNAc with 1-phenyl-3-methyl-5-pyrazolone (PMP) to enhance UV absorption, followed by reversed-phase separation and quantification at 245 nm .
- Enzymatic assays : Use GalNAc dehydrogenase coupled with NADH production, measured spectrophotometrically at 340 nm .
- Lectin-based biosensors : Immobilize GalNAc-specific lectins on gold electrodes for electrochemical impedance spectroscopy (EIS)-based detection .
Q. Advanced: How do fluorinated GalNAc analogs impact glycosylation pathways, and what synthetic strategies are used to produce them?
Fluorinated GalNAc analogs (e.g., 4-F-GalNAc) disrupt glycan biosynthesis by inhibiting glycosyltransferases or acting as metabolic chain terminators. Synthesis involves:
- Deoxyfluorination : React glucosazide or galactosazide thioglycosides with DAST (diethylaminosulfur trifluoride) to introduce fluorine at C-4 or C-6 positions .
- Protecting group strategies : Use acetyl or benzyl groups to selectively modify hydroxyls, followed by deprotection under mild acidic conditions .
- Validation : Confirm analog activity via in vitro GalNAc-T assays and cellular glycosylation profiling using glycan-specific antibodies (e.g., anti-Tn antigen) .
Q. Basic: What role does GalNAc play in bacterial polysaccharide biosynthesis, and how can its presence be confirmed in microbial capsules?
GalNAc is a key component of bacterial capsules (e.g., Acinetobacter baumannii), contributing to virulence and antibiotic resistance. Confirmation methods include:
- GC-MS analysis : Hydrolyze capsular polysaccharides with trifluoroacetic acid (TFA), derivatize with trimethylsilyl (TMS) reagents, and compare retention times to standards .
- Lectins in blotting : Use FITC-labeled Wisteria floribunda lectin for fluorescence microscopy or dot-blot assays .
- NMR spectroscopy : Identify GalNAc anomeric protons (δ 4.5–5.5 ppm) in ¹H-NMR spectra of purified polysaccharides .
Q. Advanced: How do elevated systemic GalNAc levels correlate with metabolic dysregulation, and what metabolomic approaches are used to study this?
Elevated GalNAc in serum is linked to insulin resistance and inflammation. Metabolomic workflows include:
- LC-MS/MS with isotopic labeling : Spike samples with ¹³C-GalNAc to improve quantification accuracy in human plasma .
- Pathway analysis : Integrate GalNAc data with glycoprotein biomarkers (e.g., acute-phase reactants like CRP) using tools like MetaboAnalyst .
- In vivo models: Use Galnt2 knockout mice to study GalNAc’s role in lipid metabolism and glucose homeostasis .
Q. Basic: What are the best practices for stabilizing GalNAc in cell culture media for glycosylation studies?
- Storage : Prepare fresh GalNAc solutions (50 mg/mL in PBS) and store at -20°C for ≤1 month to prevent hydrolysis .
- Serum-free conditions : Use serum-free media to avoid competing glycans during in vitro assays .
- pH control : Maintain media pH at 7.0–7.4 to minimize spontaneous deacetylation .
Q. Advanced: How can GalNAc-functionalized agarose be applied to purify lectins with distinct binding specificities?
- Column preparation : Couple GalNAc to epoxy-activated agarose at pH 9.5 (0.1 M carbonate buffer) for stable immobilization .
- Binding specificity : Screen lectins (e.g., Ricinus communis agglutinin) via elution with 0.1 M GalNAc in PBS, followed by SDS-PAGE and Coomassie staining .
- Competitive elution : Compare binding profiles with D-galactose or GlcNAc to confirm specificity .
Q. Basic: What structural features of GalNAc dictate its specificity in lectin binding?
Key determinants include:
- Axial C4 hydroxyl : Critical for hydrogen bonding with lectin pockets (e.g., Glycine max lectin) .
- N-acetyl group : Engages hydrophobic interactions in lectin binding sites (e.g., Helix pomatia agglutinin) .
- Pyranose ring conformation : β-anomers preferentially bind lectins like Vicia villosa .
Q. Advanced: What strategies resolve contradictions in GalNAc’s role in cancer progression versus immune regulation?
- Cell-type-specific studies : Compare GalNAc-T expression in tumor cells (e.g., pancreatic adenocarcinoma) versus immune cells (e.g., T-cells) via single-cell RNA-seq .
- Glycan microarray screening : Profile immune receptors (e.g., Siglecs) against synthetic GalNAc-glycoconjugates to identify pro- or anti-inflammatory roles .
- Genetic ablation : Use CRISPR/Cas9 to delete GALNT genes in murine cancer models and assess metastasis and immune infiltration .
Properties
IUPAC Name |
N-[2,4,5-trihydroxy-6-(hydroxymethyl)oxan-3-yl]acetamide | |
---|---|---|
Details | Computed by Lexichem TK 2.7.0 (PubChem release 2021.05.07) | |
Source | PubChem | |
URL | https://pubchem.ncbi.nlm.nih.gov | |
Description | Data deposited in or computed by PubChem | |
InChI |
InChI=1S/C8H15NO6/c1-3(11)9-5-7(13)6(12)4(2-10)15-8(5)14/h4-8,10,12-14H,2H2,1H3,(H,9,11) | |
Details | Computed by InChI 1.0.6 (PubChem release 2021.05.07) | |
Source | PubChem | |
URL | https://pubchem.ncbi.nlm.nih.gov | |
Description | Data deposited in or computed by PubChem | |
InChI Key |
OVRNDRQMDRJTHS-UHFFFAOYSA-N | |
Details | Computed by InChI 1.0.6 (PubChem release 2021.05.07) | |
Source | PubChem | |
URL | https://pubchem.ncbi.nlm.nih.gov | |
Description | Data deposited in or computed by PubChem | |
Canonical SMILES |
CC(=O)NC1C(C(C(OC1O)CO)O)O | |
Details | Computed by OEChem 2.3.0 (PubChem release 2021.05.07) | |
Source | PubChem | |
URL | https://pubchem.ncbi.nlm.nih.gov | |
Description | Data deposited in or computed by PubChem | |
Molecular Formula |
C8H15NO6 | |
Details | Computed by PubChem 2.1 (PubChem release 2021.05.07) | |
Source | PubChem | |
URL | https://pubchem.ncbi.nlm.nih.gov | |
Description | Data deposited in or computed by PubChem | |
DSSTOX Substance ID |
DTXSID80859634 | |
Record name | 2-Acetamido-2-deoxyhexopyranose | |
Source | EPA DSSTox | |
URL | https://comptox.epa.gov/dashboard/DTXSID80859634 | |
Description | DSSTox provides a high quality public chemistry resource for supporting improved predictive toxicology. | |
Molecular Weight |
221.21 g/mol | |
Details | Computed by PubChem 2.1 (PubChem release 2021.05.07) | |
Source | PubChem | |
URL | https://pubchem.ncbi.nlm.nih.gov | |
Description | Data deposited in or computed by PubChem | |
Solubility |
>33.2 [ug/mL] (The mean of the results at pH 7.4) | |
Record name | SID56320732 | |
Source | Burnham Center for Chemical Genomics | |
URL | https://pubchem.ncbi.nlm.nih.gov/bioassay/1996#section=Data-Table | |
Description | Aqueous solubility in buffer at pH 7.4 | |
Synthesis routes and methods
Procedure details
Retrosynthesis Analysis
AI-Powered Synthesis Planning: Our tool employs the Template_relevance Pistachio, Template_relevance Bkms_metabolic, Template_relevance Pistachio_ringbreaker, Template_relevance Reaxys, Template_relevance Reaxys_biocatalysis model, leveraging a vast database of chemical reactions to predict feasible synthetic routes.
One-Step Synthesis Focus: Specifically designed for one-step synthesis, it provides concise and direct routes for your target compounds, streamlining the synthesis process.
Accurate Predictions: Utilizing the extensive PISTACHIO, BKMS_METABOLIC, PISTACHIO_RINGBREAKER, REAXYS, REAXYS_BIOCATALYSIS database, our tool offers high-accuracy predictions, reflecting the latest in chemical research and data.
Strategy Settings
Precursor scoring | Relevance Heuristic |
---|---|
Min. plausibility | 0.01 |
Model | Template_relevance |
Template Set | Pistachio/Bkms_metabolic/Pistachio_ringbreaker/Reaxys/Reaxys_biocatalysis |
Top-N result to add to graph | 6 |
Feasible Synthetic Routes
Disclaimer and Information on In-Vitro Research Products
Please be aware that all articles and product information presented on BenchChem are intended solely for informational purposes. The products available for purchase on BenchChem are specifically designed for in-vitro studies, which are conducted outside of living organisms. In-vitro studies, derived from the Latin term "in glass," involve experiments performed in controlled laboratory settings using cells or tissues. It is important to note that these products are not categorized as medicines or drugs, and they have not received approval from the FDA for the prevention, treatment, or cure of any medical condition, ailment, or disease. We must emphasize that any form of bodily introduction of these products into humans or animals is strictly prohibited by law. It is essential to adhere to these guidelines to ensure compliance with legal and ethical standards in research and experimentation.