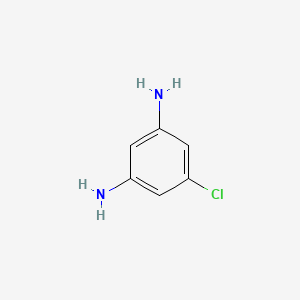
5-Chloro-m-phenylenediamine
Overview
Description
5-Chloro-m-phenylenediamine (CAS 33786-89-9) is a halogenated aromatic diamine with the molecular formula C₆H₇ClN₂ and a molecular weight of 142.59 g/mol. Structurally, it consists of a benzene ring substituted with two amino groups at the 1,3-positions and a chlorine atom at the 5-position. Key identifiers include:
- InChIKey: VZNUCJOYPXKLTA-UHFFFAOYSA-N
- SMILES: C1=C(C=C(C=C1N)N)Cl
- Synonyms: 5-Chloro-1,3-benzenediamine, this compound, 3,5-diaminochlorobenzene .
This compound is primarily utilized in organic synthesis, particularly as a precursor for dyes, pharmaceuticals, and polymers. Its chlorine substituent enhances electronic effects, influencing reactivity and stability in downstream applications .
Preparation Methods
Synthetic Routes and Reaction Conditions: 5-Chloro-m-phenylenediamine can be synthesized through several methods. One common method involves the chlorination of m-phenylenediamine. The reaction typically occurs in the presence of a chlorinating agent such as thionyl chloride or phosphorus pentachloride under controlled conditions to ensure selective chlorination at the desired position .
Industrial Production Methods: In industrial settings, the production of this compound often involves the catalytic hydrogenation of 5-chloro-1,3-dinitrobenzene. This process requires a hydrogenation catalyst, such as palladium on carbon, and is carried out under high pressure and temperature to achieve efficient reduction of the nitro groups to amine groups .
Chemical Reactions Analysis
Types of Reactions: 5-Chloro-m-phenylenediamine undergoes various chemical reactions, including:
Oxidation: The compound can be oxidized to form quinonoid structures.
Reduction: Reduction reactions can further modify the amine groups.
Substitution: The chlorine atom can be substituted with other functional groups through nucleophilic substitution reactions.
Common Reagents and Conditions:
Oxidation: Common oxidizing agents include potassium permanganate and hydrogen peroxide.
Reduction: Catalytic hydrogenation using palladium on carbon.
Substitution: Nucleophiles such as sodium methoxide or sodium ethoxide can be used for substitution reactions.
Major Products Formed:
Oxidation: Quinonoid derivatives.
Reduction: Further reduced amine derivatives.
Substitution: Various substituted derivatives depending on the nucleophile used.
Scientific Research Applications
Pharmaceutical Intermediate
5-CMPD serves as a crucial intermediate in the synthesis of various pharmaceutical compounds. Its derivatives have been studied for potential biological activities, including:
- Antimicrobial Properties: Research indicates that 5-CMPD exhibits significant antimicrobial activity against various bacterial strains, both Gram-positive and Gram-negative. The minimum inhibitory concentration (MIC) values vary, indicating effective inhibition of bacterial growth .
- Anticancer Activity: Studies have shown that 5-CMPD can induce apoptosis in cancer cell lines such as MCF-7 (breast cancer) and A549 (lung cancer), with reported IC50 values suggesting moderate cytotoxicity .
Dyes and Textiles
The compound is extensively used in the dye industry, particularly for textiles and leather. It acts as a coupling agent in hair dyes, contributing to vibrant colors and improved dye stability .
Materials Science
5-CMPD is also utilized in the production of polymers and aramid fibers, which are known for their strength and thermal stability. Its unique properties make it suitable for creating high-performance materials used in various industrial applications .
The biological activity of 5-CMPD is attributed to its ability to interact with various molecular targets, leading to the formation of reactive intermediates that can induce oxidative stress. This mechanism is critical in its antimicrobial and anticancer effects.
Antimicrobial Activity
Research has demonstrated that derivatives of 5-CMPD possess potent antibacterial properties. For instance:
- A study reported effective inhibition against multi-drug resistant bacterial strains, highlighting the potential for developing new antimicrobial agents based on this compound .
Anticancer Activity
In vivo studies involving tumor-bearing mice indicated that treatment with 5-CMPD resulted in significant tumor size reduction compared to control groups. The compound's mechanisms include inducing apoptosis and regulating the cell cycle .
Case Study 1: Anticancer Efficacy
A recent investigation assessed the effects of 5-CMPD on tumor-bearing mice, revealing a significant reduction in tumor size through mechanisms involving apoptosis and cell cycle regulation.
Case Study 2: Antimicrobial Assessment
Another study evaluated the antimicrobial efficacy of modified 5-CMPD derivatives against resistant bacterial strains, concluding that structural modifications enhanced their activity.
Comparative Analysis with Related Compounds
To understand the unique properties of 5-CMPD, it is essential to compare it with structurally related compounds:
Compound | Structure Type | Antimicrobial Activity (MIC) | Anticancer Activity (IC50) |
---|---|---|---|
This compound | Aromatic amine | Moderate | Moderate |
m-Phenylenediamine | Parent compound without chlorine | Low | Low |
o-Phenylenediamine | Isomer with amines at positions 1 & 2 | Low | Moderate |
p-Phenylenediamine | Isomer with amines at positions 1 & 4 | Low | Low |
Mechanism of Action
The mechanism of action of 5-chloro-m-phenylenediamine involves its interaction with various molecular targets. In biological systems, the compound can interact with enzymes and proteins, leading to the formation of reactive intermediates. These intermediates can cause oxidative stress and damage to cellular components, contributing to its antimicrobial and anticancer activities .
Comparison with Similar Compounds
m-Phenylenediamine (1,3-Benzenediamine)
- CAS : 108-45-2
- Formula : C₆H₈N₂
- Key Differences :
- Lacks the chlorine substituent at the 5-position.
- Lower molecular weight (108.14 g/mol vs. 142.59 g/mol).
- Reactivity: The absence of chlorine reduces steric and electronic hindrance, making it more reactive in electrophilic substitution reactions. For example, m-phenylenediamine readily forms benzimidazoles with aldehydes .
- Applications: Widely used as a dye intermediate; 5-Chloro-m-phenylenediamine’s chlorine may improve UV stability in polymer applications .
5-Chloro-o-phenylenediamine (5-Chloro-1,2-benzenediamine)
- Structure: Chlorine at the 5-position with amino groups at 1,2-positions.
- Key Differences: Ortho-diamine configuration leads to distinct reactivity. For instance, o-phenylenediamine derivatives are precursors to benzimidazoles and metal complexes . Steric hindrance between adjacent amino groups may limit applications in polymer synthesis compared to the meta isomer .
5-Chloro-4-methyl-m-phenylenediamine
- CAS : 861519-29-1
- Formula : C₇H₉ClN₂
- Key Differences :
5-Bromo-3-chloro-1,2-diaminobenzene
- CAS : 1008361-80-5
- Formula : C₆H₆BrClN₂
- Key Differences: Bromine (electronegativity 2.96) vs. chlorine (3.16) alters electronic properties. Bromine’s larger atomic radius may improve solubility in non-polar solvents .
5-Chloro-N1-phenylbenzene-1,2-diamine
- CAS : 68406-47-3
- Formula : C₁₂H₁₁ClN₂
- Key Differences: N-phenyl substitution increases molecular weight (218.69 g/mol) and steric bulk. Potential use as a monomer for polyimides, leveraging phenyl groups for thermal stability .
Table 1. Structural and Functional Comparison
Compound | CAS | Molecular Formula | Substituents | Key Applications |
---|---|---|---|---|
This compound | 33786-89-9 | C₆H₇ClN₂ | 1,3-NH₂; 5-Cl | Dyes, polymers |
m-Phenylenediamine | 108-45-2 | C₆H₈N₂ | 1,3-NH₂ | Dye intermediates |
5-Chloro-4-methyl-m-PDA | 861519-29-1 | C₇H₉ClN₂ | 1,3-NH₂; 4-CH₃; 5-Cl | Specialty chemicals |
5-Bromo-3-chloro-1,2-PDA | 1008361-80-5 | C₆H₆BrClN₂ | 1,2-NH₂; 3-Cl; 5-Br | Pharmaceutical synthesis |
5-Chloro-N1-phenyl-1,2-PDA | 68406-47-3 | C₁₂H₁₁ClN₂ | 1,2-NH₂; 5-Cl; N1-Ph | Polyimide monomers |
Biological Activity
5-Chloro-m-phenylenediamine (5-CMPD) is an aromatic amine that has garnered attention in various fields, particularly in medicinal chemistry and materials science. Its derivatives are investigated for potential biological activities , including antimicrobial and anticancer properties . This article explores the biological activity of 5-CMPD, focusing on its mechanisms of action, relevant case studies, and comparative analysis with related compounds.
Chemical Structure
This compound has the molecular formula and features a chlorine atom at the meta position relative to two amine groups on a benzene ring. This substitution significantly influences its reactivity and biological properties.
The biological activity of 5-CMPD is attributed to its ability to interact with various molecular targets, leading to the formation of reactive intermediates. These intermediates can induce oxidative stress , which is a critical factor in its antimicrobial and anticancer activities. The compound can undergo:
- Oxidation : Forming quinonoid structures that can react with cellular components.
- Reduction : Modifying the amine groups to enhance biological activity.
- Substitution : The chlorine atom allows for further chemical modifications that can improve efficacy against specific targets.
Antimicrobial Activity
Research indicates that 5-CMPD exhibits significant antimicrobial properties. A study reported that derivatives of 5-CMPD showed potent activity against various bacterial strains, including both Gram-positive and Gram-negative bacteria. The minimum inhibitory concentration (MIC) values for these compounds ranged from to , demonstrating effective inhibition of bacterial growth.
Anticancer Activity
5-CMPD has also been evaluated for its anticancer potential. In vitro studies revealed that it induces apoptosis in cancer cell lines such as MCF-7 (breast cancer) and A549 (lung cancer). The IC50 values for these cell lines were reported at approximately , indicating moderate cytotoxicity. Further research demonstrated that treatment with 5-CMPD resulted in cell cycle arrest and increased levels of pro-apoptotic markers .
Case Studies
-
Study on Anticancer Efficacy :
A recent study explored the effects of 5-CMPD on tumor-bearing mice. Results showed a significant reduction in tumor size compared to the control group, suggesting that the compound may inhibit tumor growth through mechanisms involving apoptosis and cell cycle regulation . -
Antimicrobial Assessment :
Another investigation assessed the antimicrobial efficacy of 5-CMPD derivatives against multi-drug resistant strains of bacteria. The study concluded that certain modifications to the original compound enhanced its activity, making it a candidate for developing new antimicrobial agents.
Comparative Analysis with Related Compounds
To understand the unique properties of 5-CMPD, it is essential to compare it with structurally related compounds:
Compound | Structure Type | Antimicrobial Activity (MIC) | Anticancer Activity (IC50) |
---|---|---|---|
This compound | Aromatic amine | ||
m-Phenylenediamine | Parent compound without chlorine | Moderate | Low |
o-Phenylenediamine | Isomer with amines at positions 1 & 2 | Low | Moderate |
p-Phenylenediamine | Isomer with amines at positions 1 & 4 | Low | Low |
Unique Properties
The presence of the chlorine atom in 5-CMPD enhances its reactivity compared to its non-chlorinated counterparts, allowing for greater versatility in synthetic applications and potentially improved biological activity .
Q & A
Basic Research Questions
Q. What are the standard synthetic routes for 5-Chloro-m-phenylenediamine, and how can its purity be optimized?
this compound (CAS 33786-89-9) is typically synthesized via catalytic chlorination of m-phenylenediamine or selective reduction of nitro-substituted precursors. Key steps include:
- Chlorination: Use catalytic FeCl₃ or AlCl₃ under controlled temperature (60–80°C) to minimize polychlorinated byproducts .
- Purification: Recrystallization from ethanol/water mixtures improves purity (>98%), monitored via HPLC with UV detection (λ = 254 nm) .
- Yield optimization: Adjust stoichiometry of Cl⁻ donors (e.g., Cl₂ gas or SOCl₂) to reduce side reactions. Confirm purity via melting point (mp: 135–137°C) and elemental analysis .
Q. Which spectroscopic and chromatographic methods are most effective for characterizing this compound?
- NMR spectroscopy: ¹H NMR (DMSO-d₆) shows aromatic protons at δ 6.8–7.2 ppm (meta-substitution pattern) and NH₂ signals at δ 4.5–5.0 ppm. ¹³C NMR confirms chlorine’s deshielding effect on adjacent carbons .
- Mass spectrometry: ESI-MS in positive ion mode reveals [M+H]⁺ at m/z 158.03 (C₆H₇ClN₂⁺), with fragmentation patterns distinguishing it from ortho/para isomers .
- IR spectroscopy: N-H stretches (3300–3400 cm⁻¹) and C-Cl vibrations (750–800 cm⁻¹) are diagnostic .
- HPLC: Use C18 columns with acetonitrile/water (70:30) mobile phase; retention time ~8.2 min .
Q. What safety protocols are critical when handling this compound in the lab?
- Personal protective equipment (PPE): Wear nitrile gloves, goggles, and lab coats to avoid dermal/ocular exposure. Use fume hoods to prevent inhalation .
- Waste disposal: Collect aqueous waste in sealed containers labeled “chlorinated aromatic amines” for incineration by licensed facilities .
- Emergency response: For spills, neutralize with 10% sodium bicarbonate and adsorb with vermiculite. Contaminated surfaces require rinsing with ethanol/water .
Advanced Research Questions
Q. How does this compound’s reactivity vary under oxidative vs. reductive conditions?
- Oxidation: Forms chlorinated quinones (e.g., 5-chloro-1,3-benzoquinone) using KMnO₄ in acidic media. Monitor via cyclic voltammetry (Epa ≈ 0.65 V vs. Ag/AgCl) .
- Reduction: Catalytic hydrogenation (H₂/Pd-C) yields 5-chloro-1,3-diaminocyclohexane, but over-reduction risks C-Cl bond cleavage. Control H₂ pressure (<2 atm) to retain chlorine .
- Cross-coupling: Suzuki-Miyaura reactions with aryl boronic acids require Pd(OAc)₂ and SPhos ligand for C-C bond formation at the para position .
Q. How can researchers resolve contradictions in reported solubility data for this compound?
Discrepancies in solubility (e.g., ethanol vs. DMSO) arise from crystallinity and impurities. Methodological solutions include:
- Standardized testing: Use USP dissolution apparatus (900 mL solvent, 37°C, 50 rpm). Measure saturation via UV/Vis at 280 nm .
- Purity verification: Compare batches using TLC (silica gel, ethyl acetate eluent) to detect polar impurities .
- Crystal structure analysis: XRD reveals polymorphism affecting solubility; anhydrous vs. monohydrate forms differ in hydrophilicity .
Q. What strategies optimize this compound’s stability in long-term storage for biological assays?
- Temperature: Store at –20°C under argon to prevent oxidation. Room-temperature storage in air causes 15% degradation over 30 days .
- Light sensitivity: Amber vials reduce photodegradation; UV irradiation (λ = 365 nm) induces 40% decomposition in 24 hours .
- Buffer compatibility: Avoid phosphate buffers (pH >7) due to hydrolysis; use acetate buffers (pH 4–5) for aqueous solutions .
Q. How can this compound be utilized in designing metal-organic frameworks (MOFs) or coordination polymers?
- Ligand design: The amino groups act as chelating sites for transition metals (e.g., Cu²⁺, Zn²⁺). Solvothermal synthesis (DMF, 120°C) yields MOFs with pore sizes ~8 Å .
- Applications: MOFs show promise in gas storage (CO₂ uptake: 2.5 mmol/g at 1 bar) and catalysis (e.g., Knoevenagel condensation) .
- Characterization: Analyze framework topology via PXRD and BET surface area (>500 m²/g) .
Q. What computational methods predict the electronic properties of this compound derivatives?
- DFT calculations: Use B3LYP/6-311+G(d,p) to model HOMO-LUMO gaps (ΔE ≈ 4.2 eV). Chlorine’s electron-withdrawing effect lowers HOMO energy by 0.3 eV .
- Molecular docking: Predict binding affinities to biological targets (e.g., kinase inhibitors) with AutoDock Vina; chlorine enhances hydrophobic interactions .
- MD simulations: GROMACS simulations reveal stability in lipid bilayers, suggesting membrane permeability for drug delivery studies .
Q. What advanced analytical techniques quantify trace impurities in this compound?
- GC-MS: Detect chlorinated byproducts (e.g., dichlorophenylenediamines) with DB-5MS columns; LOD ≈ 0.1 ppm .
- ICP-OES: Measure heavy metal residues (e.g., Fe, Pd) from synthesis; acceptable limits <10 ppm .
- XPS: Surface analysis confirms absence of oxidized nitrogen species (e.g., nitro groups) with N 1s binding energy ~399.5 eV .
Q. How can researchers design kinetic studies to elucidate degradation pathways of this compound in environmental matrices?
- Experimental setup: Use simulated sunlight (Xe lamp, AM 1.5G filter) and HPLC-MS to track degradation products (e.g., chlorocatechol) .
- Kinetic modeling: Apply pseudo-first-order kinetics; half-life in water (pH 7, 25°C) ≈ 14 days .
- Ecotoxicity: Test degradants on Daphnia magna (LC₅₀ <1 mg/L indicates high toxicity) .
Properties
IUPAC Name |
5-chlorobenzene-1,3-diamine | |
---|---|---|
Source | PubChem | |
URL | https://pubchem.ncbi.nlm.nih.gov | |
Description | Data deposited in or computed by PubChem | |
InChI |
InChI=1S/C6H7ClN2/c7-4-1-5(8)3-6(9)2-4/h1-3H,8-9H2 | |
Source | PubChem | |
URL | https://pubchem.ncbi.nlm.nih.gov | |
Description | Data deposited in or computed by PubChem | |
InChI Key |
VZNUCJOYPXKLTA-UHFFFAOYSA-N | |
Source | PubChem | |
URL | https://pubchem.ncbi.nlm.nih.gov | |
Description | Data deposited in or computed by PubChem | |
Canonical SMILES |
C1=C(C=C(C=C1N)Cl)N | |
Source | PubChem | |
URL | https://pubchem.ncbi.nlm.nih.gov | |
Description | Data deposited in or computed by PubChem | |
Molecular Formula |
C6H7ClN2 | |
Source | PubChem | |
URL | https://pubchem.ncbi.nlm.nih.gov | |
Description | Data deposited in or computed by PubChem | |
DSSTOX Substance ID |
DTXSID2067795 | |
Record name | 1,3-Benzenediamine, 5-chloro- | |
Source | EPA DSSTox | |
URL | https://comptox.epa.gov/dashboard/DTXSID2067795 | |
Description | DSSTox provides a high quality public chemistry resource for supporting improved predictive toxicology. | |
Molecular Weight |
142.58 g/mol | |
Source | PubChem | |
URL | https://pubchem.ncbi.nlm.nih.gov | |
Description | Data deposited in or computed by PubChem | |
CAS No. |
33786-89-9 | |
Record name | 3,5-Diaminochlorobenzene | |
Source | CAS Common Chemistry | |
URL | https://commonchemistry.cas.org/detail?cas_rn=33786-89-9 | |
Description | CAS Common Chemistry is an open community resource for accessing chemical information. Nearly 500,000 chemical substances from CAS REGISTRY cover areas of community interest, including common and frequently regulated chemicals, and those relevant to high school and undergraduate chemistry classes. This chemical information, curated by our expert scientists, is provided in alignment with our mission as a division of the American Chemical Society. | |
Explanation | The data from CAS Common Chemistry is provided under a CC-BY-NC 4.0 license, unless otherwise stated. | |
Record name | 1,3-Benzenediamine, 5-chloro- | |
Source | ChemIDplus | |
URL | https://pubchem.ncbi.nlm.nih.gov/substance/?source=chemidplus&sourceid=0033786899 | |
Description | ChemIDplus is a free, web search system that provides access to the structure and nomenclature authority files used for the identification of chemical substances cited in National Library of Medicine (NLM) databases, including the TOXNET system. | |
Record name | 1,3-Benzenediamine, 5-chloro- | |
Source | EPA Chemicals under the TSCA | |
URL | https://www.epa.gov/chemicals-under-tsca | |
Description | EPA Chemicals under the Toxic Substances Control Act (TSCA) collection contains information on chemicals and their regulations under TSCA, including non-confidential content from the TSCA Chemical Substance Inventory and Chemical Data Reporting. | |
Record name | 1,3-Benzenediamine, 5-chloro- | |
Source | EPA DSSTox | |
URL | https://comptox.epa.gov/dashboard/DTXSID2067795 | |
Description | DSSTox provides a high quality public chemistry resource for supporting improved predictive toxicology. | |
Record name | 5-CHLORO-1,3-PHENYLENEDIAMINE | |
Source | European Chemicals Agency (ECHA) | |
URL | https://echa.europa.eu/information-on-chemicals | |
Description | The European Chemicals Agency (ECHA) is an agency of the European Union which is the driving force among regulatory authorities in implementing the EU's groundbreaking chemicals legislation for the benefit of human health and the environment as well as for innovation and competitiveness. | |
Explanation | Use of the information, documents and data from the ECHA website is subject to the terms and conditions of this Legal Notice, and subject to other binding limitations provided for under applicable law, the information, documents and data made available on the ECHA website may be reproduced, distributed and/or used, totally or in part, for non-commercial purposes provided that ECHA is acknowledged as the source: "Source: European Chemicals Agency, http://echa.europa.eu/". Such acknowledgement must be included in each copy of the material. ECHA permits and encourages organisations and individuals to create links to the ECHA website under the following cumulative conditions: Links can only be made to webpages that provide a link to the Legal Notice page. | |
Synthesis routes and methods
Procedure details
Retrosynthesis Analysis
AI-Powered Synthesis Planning: Our tool employs the Template_relevance Pistachio, Template_relevance Bkms_metabolic, Template_relevance Pistachio_ringbreaker, Template_relevance Reaxys, Template_relevance Reaxys_biocatalysis model, leveraging a vast database of chemical reactions to predict feasible synthetic routes.
One-Step Synthesis Focus: Specifically designed for one-step synthesis, it provides concise and direct routes for your target compounds, streamlining the synthesis process.
Accurate Predictions: Utilizing the extensive PISTACHIO, BKMS_METABOLIC, PISTACHIO_RINGBREAKER, REAXYS, REAXYS_BIOCATALYSIS database, our tool offers high-accuracy predictions, reflecting the latest in chemical research and data.
Strategy Settings
Precursor scoring | Relevance Heuristic |
---|---|
Min. plausibility | 0.01 |
Model | Template_relevance |
Template Set | Pistachio/Bkms_metabolic/Pistachio_ringbreaker/Reaxys/Reaxys_biocatalysis |
Top-N result to add to graph | 6 |
Feasible Synthetic Routes
Disclaimer and Information on In-Vitro Research Products
Please be aware that all articles and product information presented on BenchChem are intended solely for informational purposes. The products available for purchase on BenchChem are specifically designed for in-vitro studies, which are conducted outside of living organisms. In-vitro studies, derived from the Latin term "in glass," involve experiments performed in controlled laboratory settings using cells or tissues. It is important to note that these products are not categorized as medicines or drugs, and they have not received approval from the FDA for the prevention, treatment, or cure of any medical condition, ailment, or disease. We must emphasize that any form of bodily introduction of these products into humans or animals is strictly prohibited by law. It is essential to adhere to these guidelines to ensure compliance with legal and ethical standards in research and experimentation.