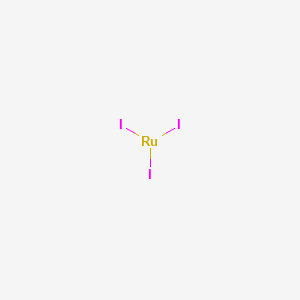
Ruthenium iodide
- Click on QUICK INQUIRY to receive a quote from our team of experts.
- With the quality product at a COMPETITIVE price, you can focus more on your research.
Overview
Description
Ruthenium iodide is a chemical compound consisting of ruthenium and iodine, with the chemical formula RuI₃. It is a black solid that is sparingly soluble in water.
Mechanism of Action
Target of Action
Ruthenium iodide, a chemical compound containing ruthenium and iodine, has been studied for its potential anticancer properties . The primary targets of this compound are cancer cells, particularly those of the MCF-7 and HeLa lines . It has been suggested that this compound interacts with plasma proteins, particularly serum albumin and transferrin, and binds to nucleic acids .
Mode of Action
This compound interacts with its targets through a process known as immunogenic cell death (ICD) . This process makes dying cancer cells ‘visible’ to the immune system, initiating a prolonged immune response against the tumor . The interaction of this compound with cancer cells leads to the release of reactive oxygen species intracellularly, which could indicate that they are involved in cell apoptosis .
Biochemical Pathways
The biochemical pathways affected by this compound are primarily related to cancer cell apoptosis . The compound’s interaction with cancer cells triggers the release of reactive oxygen species, which can cause damage to biomolecules such as DNA . This damage can lead to cell apoptosis, a form of programmed cell death .
Pharmacokinetics
It is known that this compound is sparingly soluble in water , which may impact its bioavailability.
Result of Action
The result of this compound’s action is the induction of apoptosis in cancer cells . This is achieved through the compound’s interaction with plasma proteins and nucleic acids, leading to the release of reactive oxygen species and subsequent damage to biomolecules . This damage triggers cell apoptosis, leading to the death of cancer cells .
Action Environment
The action of this compound can be influenced by environmental factors. For instance, the compound’s solubility in water may affect its bioavailability and, consequently, its efficacy . Additionally, this compound is a black solid , and its physical state may also influence its stability and action
Biochemical Analysis
Biochemical Properties
Ruthenium iodide plays a crucial role in biochemical reactions due to its ability to interact with a variety of enzymes, proteins, and other biomolecules. It readily forms coordination complexes, which can influence the activity of these biomolecules. For instance, this compound has been shown to interact with DNA, leading to the formation of DNA adducts that can inhibit DNA replication and transcription . Additionally, it can bind to proteins such as albumin and apotransferrin, affecting their function and stability . These interactions are primarily driven by the ability of this compound to adopt multiple oxidation states, allowing it to participate in redox reactions and other biochemical processes .
Cellular Effects
This compound has been observed to exert significant effects on various types of cells and cellular processes. In cancer cells, for example, this compound complexes have demonstrated cytotoxic activity, leading to cell apoptosis through the generation of reactive oxygen species . This compound can also influence cell signaling pathways, gene expression, and cellular metabolism. By interacting with key signaling molecules and transcription factors, this compound can modulate the expression of genes involved in cell proliferation, differentiation, and survival . Furthermore, its impact on cellular metabolism includes alterations in metabolic flux and changes in the levels of various metabolites .
Molecular Mechanism
The molecular mechanism of action of this compound involves several key processes. At the molecular level, this compound can bind to DNA, forming stable adducts that inhibit DNA replication and transcription . This binding interaction is facilitated by the ability of this compound to intercalate between DNA bases, disrupting the helical structure of the DNA molecule. Additionally, this compound can inhibit or activate enzymes by binding to their active sites or allosteric sites, thereby modulating their activity . Changes in gene expression induced by this compound are often a result of its interaction with transcription factors and other regulatory proteins .
Temporal Effects in Laboratory Settings
In laboratory settings, the effects of this compound can change over time due to its stability and degradation. Studies have shown that this compound complexes are relatively stable in solution, with minimal degradation observed over extended periods . The long-term effects of this compound on cellular function can vary depending on the specific experimental conditions. For instance, prolonged exposure to this compound may lead to sustained changes in gene expression and cellular metabolism, which can have lasting impacts on cell function .
Dosage Effects in Animal Models
The effects of this compound in animal models are dose-dependent, with varying outcomes observed at different dosages. At low doses, this compound may exhibit minimal toxicity and exert beneficial effects, such as inhibiting tumor growth in cancer models . At higher doses, this compound can induce toxic effects, including oxidative stress, organ damage, and adverse effects on overall health . Threshold effects have also been observed, where a certain dosage is required to achieve a therapeutic effect, beyond which toxicity may occur .
Metabolic Pathways
This compound is involved in several metabolic pathways, interacting with various enzymes and cofactors. It can participate in redox reactions, influencing the levels of reactive oxygen species and other metabolites . Additionally, this compound can affect metabolic flux by modulating the activity of key enzymes involved in metabolic pathways . These interactions can lead to changes in the levels of metabolites, impacting cellular metabolism and overall cell function .
Transport and Distribution
Within cells and tissues, this compound is transported and distributed through interactions with transporters and binding proteins. For example, it can bind to albumin and apotransferrin, facilitating its transport in the bloodstream and its distribution to various tissues . The localization and accumulation of this compound within cells can also be influenced by its interactions with specific transporters and binding proteins .
Subcellular Localization
The subcellular localization of this compound is an important factor in determining its activity and function. This compound can be directed to specific compartments or organelles within the cell through targeting signals and post-translational modifications . For instance, it may accumulate in the nucleus, where it can interact with DNA and other nuclear proteins, or in the mitochondria, where it can influence cellular metabolism and energy production . The specific localization of this compound can have significant implications for its biochemical and cellular effects.
Preparation Methods
Ruthenium iodide can be synthesized through several methods:
Reaction with Hydroiodic Acid: Ruthenium tetroxide reacts with aqueous hydroiodic acid to form this compound[ \text{RuO}_4 + \text{excess HI} \rightarrow \text{RuI}_3 ]
Thermal Decomposition: Pentaamminethis compound decomposes thermally to yield this compound and ammonia[ \text{Ru(NH}_3\text{)}_5\text{I}_3 \rightarrow \text{RuI}_3 + 5 \text{NH}_3 ]
Salt Metathesis: Hydrated ruthenium chloride reacts with potassium iodide in aqueous solution[ \text{RuCl}_3 \cdot x\text{H}_2\text{O} + 3 \text{KI} \rightarrow \text{RuI}_3 + 3 \text{KCl} + x\text{H}_2\text{O} ]
Chemical Reactions Analysis
Ruthenium iodide undergoes several types of chemical reactions:
Oxidation: this compound can be oxidized to form higher oxidation state compounds.
Reduction: It can be reduced to lower oxidation state compounds.
Substitution: this compound can participate in substitution reactions where iodine atoms are replaced by other ligands.
Complex Formation: It can form complexes with various ligands, enhancing its reactivity and applications.
Common reagents used in these reactions include hydroiodic acid, potassium iodide, and various ligands. The major products formed depend on the specific reaction conditions and reagents used .
Scientific Research Applications
Ruthenium iodide has several scientific research applications:
Catalysis: It is used as a catalyst in various chemical reactions, including hydrogenation and oxidation reactions.
Materials Science: this compound is used in the preparation of thin films and coatings through chemical vapor deposition techniques.
Medicine: Ruthenium compounds, including this compound, are being explored for their potential anticancer properties and other medicinal applications.
Anion Sensing: This compound complexes are used in the development of luminescent chemosensors for detecting anions
Comparison with Similar Compounds
Ruthenium iodide can be compared with other ruthenium halides such as ruthenium chloride and ruthenium bromide. While all these compounds share similar properties, this compound is unique in its specific reactivity and applications. Similar compounds include:
Ruthenium Chloride (RuCl₃): Used in catalysis and materials science.
Ruthenium Bromide (RuBr₃): Similar applications to this compound but with different reactivity.
Osmium Iodide (OsI₃): Shares similar properties with this compound but is less commonly used .
Properties
CAS No. |
13896-65-6 |
---|---|
Molecular Formula |
I3Ru |
Molecular Weight |
481.8 g/mol |
IUPAC Name |
ruthenium(3+);triiodide |
InChI |
InChI=1S/3HI.Ru/h3*1H;/q;;;+3/p-3 |
InChI Key |
LJZVDOUZSMHXJH-UHFFFAOYSA-K |
SMILES |
[Ru](I)(I)I |
Canonical SMILES |
[Ru+3].[I-].[I-].[I-] |
Origin of Product |
United States |
Q1: What makes ruthenium iodide complexes particularly interesting for olefin metathesis reactions?
A1: this compound complexes, unlike their chloride counterparts, exhibit remarkable selectivity in olefin metathesis reactions. [, ] For instance, cis-diiodo sulfur chelated ruthenium benzylidenes demonstrate inactivity towards strained cycloalkenes and internal olefins, yet readily catalyze metathesis reactions involving terminal dienes. [] This unique selectivity stems from the steric bulk of the iodide ligands, hindering the approach of bulky substrates like norbornenes. []
Q2: Beyond olefin metathesis, what other catalytic applications have been explored for this compound complexes?
A3: this compound compounds have demonstrated promising catalytic activity in N-alkylation reactions. [] Studies comparing ruthenium-arene compounds with chloride, bromide, or iodide ligands revealed that the this compound complexes exhibited the highest catalytic activities in the redox-neutral alkylation of tert-butylamine with phenethyl alcohol. []
Q3: Have there been any computational studies to understand the reactivity of these complexes?
A4: Yes, Density Functional Theory (DFT) calculations have been employed to gain insights into the reactivity and selectivity of this compound catalysts in olefin metathesis reactions. [] These calculations provide a theoretical basis for understanding the observed experimental results and guide further development of novel catalysts with tailored properties.
Q4: What is the significance of synthesizing and characterizing heterometallic this compound compounds?
A5: The synthesis and structural characterization of heterometallic compounds like Pr4I5Ru and La4I5Ru contribute to a broader understanding of metal-metal bonding and cluster formation. [, ] These compounds, featuring ruthenium-centered clusters within their structures, provide valuable insights into the influence of different metal combinations on structural properties and potential applications. []
Q5: How does the choice of lanthanide metal impact the structure of this compound cluster compounds?
A6: Studies on compounds like Pr3I3Ru and Y3I3Ru highlight the influence of the lanthanide metal on the structural distortions within chains of centered metal clusters. [] The varying ionic radii and electronic configurations of lanthanides like praseodymium and yttrium lead to distinct structural arrangements, influencing the overall properties of these materials. []
Disclaimer and Information on In-Vitro Research Products
Please be aware that all articles and product information presented on BenchChem are intended solely for informational purposes. The products available for purchase on BenchChem are specifically designed for in-vitro studies, which are conducted outside of living organisms. In-vitro studies, derived from the Latin term "in glass," involve experiments performed in controlled laboratory settings using cells or tissues. It is important to note that these products are not categorized as medicines or drugs, and they have not received approval from the FDA for the prevention, treatment, or cure of any medical condition, ailment, or disease. We must emphasize that any form of bodily introduction of these products into humans or animals is strictly prohibited by law. It is essential to adhere to these guidelines to ensure compliance with legal and ethical standards in research and experimentation.