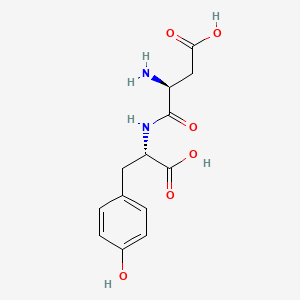
Asp-tyr
Overview
Description
Aspartic acid-tyrosine, commonly referred to as Asp-Tyr, is a dipeptide composed of the amino acids aspartic acid and tyrosine. This compound is of significant interest in various fields of scientific research due to its unique properties and potential applications. This compound is known for its role in modulating metabolic pathways and its potential therapeutic benefits.
Mechanism of Action
Target of Action
The primary targets of Asp-Tyr are various types of bacteria, including Escherichia coli, Pseudomonas aeruginosa, and Monilia albicans. This compound has been shown to have antimicrobial effects on these bacteria . .
Mode of Action
This compound interacts with its bacterial targets by binding to the phospholipid head group of the bacterial cell membrane. The N-terminal of this compound first binds to the phospholipid head group, while its C-terminal amino acid residue binds the hydrophobic tail, thereby creating a gap in the membrane when the phospholipids are clustered by hydrogen bonding . This interaction leads to changes in the bacterial cell membrane, causing damage and content leakage .
Biochemical Pathways
The action of this compound affects the membrane transport and amino acid metabolism pathways of the targeted bacteria . By disrupting the bacterial cell membrane, this compound interferes with the normal functioning of these pathways, leading to the death of the bacteria .
Pharmacokinetics
The minimum inhibitory concentration (mic) of this compound againstE. coli has been reported to be 18.075 mg/mL This suggests that this compound can be effective at relatively high concentrations
Result of Action
The action of this compound results in the death of the targeted bacteria. Specifically, this compound causes damage to the bacterial cell membrane and leads to content leakage . This disrupts the normal functioning of the bacteria and ultimately leads to their death .
Action Environment
The action of this compound can be influenced by the environment in which it is present. For example, the effects of a hydrophobic environment on the secondary structures of this compound have been studied using circular dichroism and atomic force microscopy
Biochemical Analysis
Biochemical Properties
Asp-Tyr is involved in numerous biochemical reactions. The Arabidopsis Protein Kinase SOS2, which functions in salt tolerance, can be activated by a serine-156 or tyrosine-175 to aspartic acid mutation within the activation loop . This highlights the critical role of this compound in the regulation of protein kinases and the biochemical properties of SOS2 .
Cellular Effects
The effects of this compound on various types of cells and cellular processes are profound. For instance, the peptide DDDY, which contains this compound, affects genes involved in Pseudomonas aeruginosa membrane transport and amino acid metabolism pathways . This demonstrates how this compound influences cell function, including impacts on cell signaling pathways, gene expression, and cellular metabolism.
Molecular Mechanism
The molecular mechanism of this compound involves binding interactions with biomolecules, enzyme inhibition or activation, and changes in gene expression. For example, the activation of the SOS2 protein kinase by this compound involves an intramolecular reaction mechanism of SOS2 autophosphorylation .
Preparation Methods
Synthetic Routes and Reaction Conditions
Asp-Tyr can be synthesized using standard peptide synthesis techniques. One common method involves the use of solid-phase peptide synthesis (SPPS), where the amino acids are sequentially added to a growing peptide chain anchored to a solid resin. The synthesis typically begins with the attachment of aspartic acid to the resin, followed by the coupling of tyrosine using a coupling reagent such as N,N’-diisopropylcarbodiimide (DIC) and hydroxybenzotriazole (HOBt). The reaction is carried out under mild conditions to prevent racemization and degradation of the amino acids.
Industrial Production Methods
In an industrial setting, the production of this compound may involve large-scale SPPS or solution-phase synthesis. The choice of method depends on the desired scale and purity of the final product. Industrial production often employs automated peptide synthesizers to ensure consistency and efficiency. The synthesized dipeptide is then purified using techniques such as high-performance liquid chromatography (HPLC) to achieve the required purity levels.
Chemical Reactions Analysis
Types of Reactions
Asp-Tyr undergoes various chemical reactions, including:
Oxidation: The tyrosine residue can be oxidized to form dopaquinone, which can further react to form melanin-like compounds.
Reduction: Reduction reactions can target the carboxyl groups of aspartic acid, converting them to alcohols.
Substitution: The phenolic hydroxyl group of tyrosine can undergo electrophilic substitution reactions, such as nitration or sulfonation.
Common Reagents and Conditions
Oxidation: Hydrogen peroxide or periodate can be used as oxidizing agents under acidic or neutral conditions.
Reduction: Sodium borohydride or lithium aluminum hydride are common reducing agents used under mild conditions.
Substitution: Nitration can be achieved using nitric acid in the presence of sulfuric acid, while sulfonation can be carried out using sulfur trioxide or chlorosulfonic acid.
Major Products Formed
Oxidation: Dopaquinone and melanin-like compounds.
Reduction: Reduced forms of aspartic acid derivatives.
Substitution: Nitrated or sulfonated tyrosine derivatives.
Scientific Research Applications
Asp-Tyr has a wide range of applications in scientific research:
Chemistry: Used as a model compound to study peptide synthesis and reactions.
Biology: Investigated for its role in metabolic pathways and as a regulatory molecule in cellular processes.
Medicine: Explored for its potential therapeutic effects, including antioxidant properties and modulation of glucose metabolism.
Industry: Utilized in the development of peptide-based drugs and as a component in biochemical assays.
Comparison with Similar Compounds
Asp-Tyr is unique among dipeptides due to its specific inhibitory effect on GAPC and its role in modulating glucose metabolism. Similar compounds include other dipeptides such as:
Glycine-tyrosine: Known for its role in neurotransmission.
Valine-tyrosine-valine: Studied for its potential in enhancing muscle growth.
Methionine-enkephalin: A pentapeptide with opioid-like activity.
These compounds differ in their biological activities and target pathways, highlighting the unique properties of this compound in metabolic regulation.
Properties
IUPAC Name |
(3S)-3-amino-4-[[(1S)-1-carboxy-2-(4-hydroxyphenyl)ethyl]amino]-4-oxobutanoic acid | |
---|---|---|
Source | PubChem | |
URL | https://pubchem.ncbi.nlm.nih.gov | |
Description | Data deposited in or computed by PubChem | |
InChI |
InChI=1S/C13H16N2O6/c14-9(6-11(17)18)12(19)15-10(13(20)21)5-7-1-3-8(16)4-2-7/h1-4,9-10,16H,5-6,14H2,(H,15,19)(H,17,18)(H,20,21)/t9-,10-/m0/s1 | |
Source | PubChem | |
URL | https://pubchem.ncbi.nlm.nih.gov | |
Description | Data deposited in or computed by PubChem | |
InChI Key |
NALWOULWGHTVDA-UWVGGRQHSA-N | |
Source | PubChem | |
URL | https://pubchem.ncbi.nlm.nih.gov | |
Description | Data deposited in or computed by PubChem | |
Canonical SMILES |
C1=CC(=CC=C1CC(C(=O)O)NC(=O)C(CC(=O)O)N)O | |
Source | PubChem | |
URL | https://pubchem.ncbi.nlm.nih.gov | |
Description | Data deposited in or computed by PubChem | |
Isomeric SMILES |
C1=CC(=CC=C1C[C@@H](C(=O)O)NC(=O)[C@H](CC(=O)O)N)O | |
Source | PubChem | |
URL | https://pubchem.ncbi.nlm.nih.gov | |
Description | Data deposited in or computed by PubChem | |
Molecular Formula |
C13H16N2O6 | |
Source | PubChem | |
URL | https://pubchem.ncbi.nlm.nih.gov | |
Description | Data deposited in or computed by PubChem | |
DSSTOX Substance ID |
DTXSID20177389 | |
Record name | Aspartyltyrosine | |
Source | EPA DSSTox | |
URL | https://comptox.epa.gov/dashboard/DTXSID20177389 | |
Description | DSSTox provides a high quality public chemistry resource for supporting improved predictive toxicology. | |
Molecular Weight |
296.28 g/mol | |
Source | PubChem | |
URL | https://pubchem.ncbi.nlm.nih.gov | |
Description | Data deposited in or computed by PubChem | |
CAS No. |
22840-03-5 | |
Record name | L-α-Aspartyl-L-tyrosine | |
Source | CAS Common Chemistry | |
URL | https://commonchemistry.cas.org/detail?cas_rn=22840-03-5 | |
Description | CAS Common Chemistry is an open community resource for accessing chemical information. Nearly 500,000 chemical substances from CAS REGISTRY cover areas of community interest, including common and frequently regulated chemicals, and those relevant to high school and undergraduate chemistry classes. This chemical information, curated by our expert scientists, is provided in alignment with our mission as a division of the American Chemical Society. | |
Explanation | The data from CAS Common Chemistry is provided under a CC-BY-NC 4.0 license, unless otherwise stated. | |
Record name | Aspartyltyrosine | |
Source | ChemIDplus | |
URL | https://pubchem.ncbi.nlm.nih.gov/substance/?source=chemidplus&sourceid=0022840035 | |
Description | ChemIDplus is a free, web search system that provides access to the structure and nomenclature authority files used for the identification of chemical substances cited in National Library of Medicine (NLM) databases, including the TOXNET system. | |
Record name | Aspartyltyrosine | |
Source | EPA DSSTox | |
URL | https://comptox.epa.gov/dashboard/DTXSID20177389 | |
Description | DSSTox provides a high quality public chemistry resource for supporting improved predictive toxicology. | |
Retrosynthesis Analysis
AI-Powered Synthesis Planning: Our tool employs the Template_relevance Pistachio, Template_relevance Bkms_metabolic, Template_relevance Pistachio_ringbreaker, Template_relevance Reaxys, Template_relevance Reaxys_biocatalysis model, leveraging a vast database of chemical reactions to predict feasible synthetic routes.
One-Step Synthesis Focus: Specifically designed for one-step synthesis, it provides concise and direct routes for your target compounds, streamlining the synthesis process.
Accurate Predictions: Utilizing the extensive PISTACHIO, BKMS_METABOLIC, PISTACHIO_RINGBREAKER, REAXYS, REAXYS_BIOCATALYSIS database, our tool offers high-accuracy predictions, reflecting the latest in chemical research and data.
Strategy Settings
Precursor scoring | Relevance Heuristic |
---|---|
Min. plausibility | 0.01 |
Model | Template_relevance |
Template Set | Pistachio/Bkms_metabolic/Pistachio_ringbreaker/Reaxys/Reaxys_biocatalysis |
Top-N result to add to graph | 6 |
Feasible Synthetic Routes
Disclaimer and Information on In-Vitro Research Products
Please be aware that all articles and product information presented on BenchChem are intended solely for informational purposes. The products available for purchase on BenchChem are specifically designed for in-vitro studies, which are conducted outside of living organisms. In-vitro studies, derived from the Latin term "in glass," involve experiments performed in controlled laboratory settings using cells or tissues. It is important to note that these products are not categorized as medicines or drugs, and they have not received approval from the FDA for the prevention, treatment, or cure of any medical condition, ailment, or disease. We must emphasize that any form of bodily introduction of these products into humans or animals is strictly prohibited by law. It is essential to adhere to these guidelines to ensure compliance with legal and ethical standards in research and experimentation.