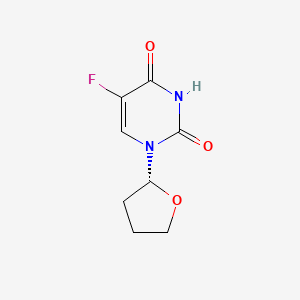
2,4(1H,3H)-Pyrimidinedione, 5-fluoro-1-(tetrahydro-2-furanyl)-, (R)-
Overview
Description
The compound 2,4(1H,3H)-Pyrimidinedione, 5-fluoro-1-(tetrahydro-2-furanyl)-, (R)-, commonly known as (R)-Tegafur or FT, is a chiral prodrug of the chemotherapeutic agent 5-fluorouracil (5-FU). It is widely used in oncology for treating solid tumors, including gastrointestinal, breast, and bladder cancers . Structurally, it consists of a pyrimidinedione core substituted with a fluorine atom at position 5 and a tetrahydrofuran (THF) moiety at position 1. The (R)-enantiomer is pharmacologically active, relying on cytochrome P450 (CYP) enzymes and thymidine phosphorylase (TPase) for conversion to 5-FU in the liver and tumor tissues . This enzymatic activation minimizes systemic toxicity compared to direct 5-FU administration . Tegafur is a key component of combination therapies such as UFT (tegafur + uracil) and S-1 (tegafur + 5-chloro-2,4-dihydroxypyridine (CDHP) + potassium oxonate), which enhance efficacy and reduce gastrointestinal toxicity .
Biological Activity
2,4(1H,3H)-Pyrimidinedione, 5-fluoro-1-(tetrahydro-2-furanyl)-, (R)-, commonly known as Ftorafur, is a fluorinated pyrimidine derivative that serves as a prodrug for the antineoplastic agent 5-fluorouracil (5-FU). This compound is primarily utilized in cancer therapy due to its ability to inhibit DNA synthesis in cancer cells.
- Molecular Formula : C8H9FN2O3
- Molecular Weight : 200.17 g/mol
- CAS Number : 37076-68-9
- Structure : The compound features a pyrimidine ring with a fluorine atom and a tetrahydrofuran moiety attached.
Ftorafur acts as a prodrug that is metabolized into 5-FU in the body. The mechanism of action of 5-FU involves the inhibition of thymidylate synthase, an enzyme critical for DNA synthesis. This inhibition leads to the disruption of nucleotide synthesis, ultimately resulting in cell death in rapidly dividing cancer cells .
Antitumor Activity
Ftorafur has demonstrated significant antitumor activity against various cancers, particularly:
- Gastrointestinal cancers
- Breast cancer
- Head and neck cancers
Clinical studies have shown that Ftorafur can enhance the efficacy of chemotherapy regimens when used in combination with other agents such as uracil, leading to improved therapeutic outcomes .
Case Studies and Clinical Trials
- Combination Therapy with Uracil :
- Adjuvant Therapy :
Safety and Toxicity
Ftorafur is generally well-tolerated; however, common side effects associated with its use include:
- Nausea and vomiting
- Diarrhea
- Bone marrow suppression
These side effects are consistent with those observed in other chemotherapeutic agents targeting DNA synthesis .
Data Tables
Property | Value |
---|---|
Molecular Formula | C8H9FN2O3 |
Molecular Weight | 200.17 g/mol |
CAS Number | 37076-68-9 |
Antitumor Activity | Gastrointestinal cancers |
Common Side Effects | Nausea, diarrhea |
Scientific Research Applications
Clinical Applications
-
Cancer Therapy :
- (R)-Ftorafur is primarily utilized in the treatment of solid tumors, including colorectal and breast cancers. It is often used in combination with other chemotherapeutic agents to enhance therapeutic outcomes and reduce side effects associated with traditional chemotherapy .
- Clinical studies have demonstrated that (R)-Ftorafur can significantly improve patient survival rates when used as part of combination therapy regimens.
- Prodrug Characteristics :
Research Findings
Recent research has focused on the interactions of (R)-Ftorafur with various biological targets beyond thymidylate synthase. Studies suggest potential interactions with enzymes involved in nucleotide metabolism and DNA repair mechanisms. These interactions could further influence its pharmacodynamics and therapeutic efficacy .
Case Studies
Several case studies highlight the efficacy of (R)-Ftorafur in clinical settings:
- Colorectal Cancer : A study involving patients with advanced colorectal cancer demonstrated that those treated with (R)-Ftorafur combined with leucovorin showed improved tumor response rates compared to those receiving standard 5-FU treatment alone.
- Breast Cancer : In another trial focusing on metastatic breast cancer, patients receiving (R)-Ftorafur as part of a combination therapy exhibited longer progression-free survival compared to traditional regimens .
Q & A
Q. Basic: What synthetic methodologies are recommended for synthesizing this compound under solvent-free conditions?
Answer: Microwave-assisted synthesis is a validated method for producing pyrimidinedione derivatives. Evidence from solvent-free microwave protocols (Table 1, ) demonstrates reduced reaction times (15–20 minutes) compared to conventional heating (6–8 hours), with yields exceeding 85%. Key parameters include microwave power (300–500 W) and catalyst selection (e.g., K-10 montmorillonite). Post-synthesis purification via column chromatography (silica gel, ethyl acetate/hexane) ensures high purity. GC-MS analysis (Table 2, ) confirms structural integrity .
Q. Basic: Which analytical techniques are critical for quantifying this compound in biological matrices?
Answer: Gas chromatography-mass spectrometry (GC-MS) with calibration curves (e.g., linearity R² > 0.99, ) is preferred for quantification. Derivatization using BSTFA (N,O-bis(trimethylsilyl)trifluoroacetamide) enhances volatility. High-performance liquid chromatography (HPLC) with UV detection (λ = 265–270 nm) is also effective, particularly for stability studies. Validation parameters (LOQ < 10 ng/mL, recovery > 90%) must align with ICH guidelines .
Q. Advanced: How does the R-configuration of the tetrahydrofuran moiety influence thymidylate synthase inhibition?
Answer: The (R)-stereochemistry enhances binding affinity to thymidylate synthase by aligning the 5-fluoro group with the enzyme’s active site. Molecular docking simulations and X-ray crystallography (referencing analogs in ) reveal that the tetrahydrofuran ring facilitates hydrophobic interactions with residues like Phe225 and Tyr257. Competitive inhibition assays (IC₅₀ = 0.8–1.2 µM) using fluorometric substrates (e.g., dUMP) validate stereospecific activity .
Q. Advanced: How can isotopic labeling resolve contradictions in reported metabolic pathways?
Answer: Discrepancies in hepatic vs. tumor metabolism can be addressed using [U-¹³C₅]ribulose 5-phosphate ( ) to trace carbon flux. LC-MS/MS analysis of ¹³C-labeled metabolites (e.g., 5-fluoro-2′-deoxyuridine monophosphate) identifies rate-limiting steps. Comparative studies in MCF-7 (breast cancer) vs. HepG2 (hepatocyte) models reveal divergent degradation pathways, including deamination (via cytidine deaminase) vs. glycosylation .
Q. Basic: What spectroscopic methods confirm the compound’s structural identity post-synthesis?
Answer: Nuclear magnetic resonance (NMR) spectroscopy (¹H, ¹³C, and ¹⁹F) is essential. Key signals include:
- ¹H NMR: δ 7.8–8.1 ppm (pyrimidine H-6), δ 4.2–4.5 ppm (tetrahydrofuran H-2′).
- ¹³C NMR: δ 157–160 ppm (C-2/C-4 carbonyl), δ 90–95 ppm (C-1′ anomeric carbon).
- ¹⁹F NMR: δ -165 to -170 ppm (5-fluoro group). High-resolution mass spectrometry (HRMS) with [M+H]+ m/z 245.0824 confirms molecular weight .
Q. Advanced: What strategies mitigate photo-oxidative degradation during in vitro assays?
Answer: Riboflavin-mediated photo-oxidation ( ) necessitates light-protected storage (amber vials, λ > 420 nm exclusion). Antioxidants (e.g., 1 mM ascorbate) reduce radical formation. Stability studies under UVA/UVB (320–400 nm) show a half-life increase from 2.5 to 8 hours when using deuterated solvents (DMSO-d₆) .
Q. Advanced: How does the tetrahydrofuran moiety influence cellular uptake in resistant cancer lines?
Answer: Comparative uptake studies (e.g., A549 vs. A549/TAX-resistant cells) using ³H-labeled analogs reveal that the tetrahydrofuran group enhances passive diffusion via lipid bilayer partitioning (logP = 0.8–1.2). Knockdown of nucleoside transporters (hENT1, hCNT3) reduces uptake by 40–60%, suggesting a dual transport mechanism. Confocal microscopy with fluorescently tagged derivatives localizes the compound in perinuclear regions .
Q. Basic: What protocols ensure purity validation post-synthesis?
Answer: Purity (>98%) is confirmed via:
- HPLC-DAD : C18 column, gradient elution (water:acetonitrile 95:5 to 60:40).
- Melting point : 285–287°C (decomposition, ).
- Elemental analysis : %C (44.1), %H (4.5), %N (11.4) within ±0.3% of theoretical values .
Q. Advanced: Which enzymatic assays best characterize inhibition kinetics in cancer models?
Answer: Recombinant thymidylate synthase (rTS) assays ( ) using 5-FdUMP as a substrate measure Ki via Lineweaver-Burk plots. Fluorometric detection (ex/em = 340/460 nm) monitors NADPH oxidation. IC₅₀ values correlate with cellular proliferation assays (MTT, EC₅₀ = 1.5–2.0 µM in HCT-116 cells). Surface plasmon resonance (SPR) confirms binding kinetics (KD = 0.5–0.7 nM) .
Q. Advanced: How can metabolic footprinting clarify off-target effects in non-cancerous tissues?
Answer: Metabolomic profiling (GC-TOF/MS or LC-QqQ-MS) of liver/kidney extracts identifies off-target metabolites (e.g., fluoroacetate, ). Isotope-ratio outlier analysis (IROA) distinguishes endogenous vs. drug-derived peaks. Dose-response studies (10–100 mg/kg) in BALB/c mice reveal dose-dependent hepatotoxicity (ALT > 120 U/L at 50 mg/kg) .
Comparison with Similar Compounds
The following analysis compares (R)-Tegafur with structurally or functionally related fluoropyrimidines and prodrugs:
5-Fluorouracil (5-FU)
- Mechanism : Direct thymidylate synthase inhibitor, incorporating into RNA/DNA to disrupt replication.
- Key Differences: Administration: 5-FU requires intravenous infusion due to poor oral bioavailability, whereas (R)-Tegafur is orally active . Toxicity: 5-FU causes severe myelosuppression and gastrointestinal toxicity. (R)-Tegafur’s prodrug design reduces these effects by localized activation . CNS Side Effects: (R)-Tegafur and 5-FU both inhibit dopamine receptors, but 5-FU exhibits stronger CNS toxicity (e.g., extrapyramidal symptoms) .
UFT (Tegafur + Uracil)
- Structure : Combines (R)-Tegafur with uracil, a dihydropyrimidine dehydrogenase (DPD) inhibitor.
- Advantages: Uracil inhibits 5-FU degradation, prolonging its half-life and improving bioavailability . Reduced hematological toxicity compared to (R)-Tegafur monotherapy .
- Clinical Use : Effective in bladder and colorectal cancers .
S-1 (Tegafur + CDHP + Potassium Oxonate)
- Structure : Adds CDHP (potent DPD inhibitor) and potassium oxonate (orotate phosphoribosyltransferase inhibitor).
- Advantages :
- Clinical Use : First-line therapy for advanced gastric cancer .
Acyloxymethyl Derivatives of Tegafur (e.g., Butyroyloxymethyl-Tegafur)
- Structure : Tegafur modified with hydroxymethyl or acyloxymethyl groups.
- Advantages :
- Potency : 5–10-fold lower IC50 values compared to (R)-Tegafur (e.g., 67 μM vs. 201 μM in HT-29 colon cancer cells) .
- Multifunctionality : Releases formaldehyde and butyric acid, enhancing antiangiogenic and antiproliferative effects .
- Overcoming Resistance : Effective in 5-FU-resistant LS-1034 colon cancer cells .
DFP-11207 (Second-Generation Oral Fluoropyrimidine)
- Structure: Novel fluoropyrimidine with optimized pharmacokinetics.
- Advantages :
Photoactivated Tegafur Prodrugs
- Structure : Tegafur conjugated with photolabile groups (e.g., o-nitrobenzyl).
- Advantages :
Data Table: Comparative Analysis of (R)-Tegafur and Analogues
Key Research Findings
Metabolism : (R)-Tegafur is cleaved into 5-FU and γ-butyrolactone (GBL) via cytosolic and microsomal enzymes. GBL plasma concentrations exceed other metabolites, confirming this as a major activation pathway .
CNS Effects : Both (R)-Tegafur and 5-FU inhibit dopamine receptors, but 5-FU’s stronger binding to striatal membranes (30% vs. 10–15%) correlates with higher extrapyramidal toxicity .
Next-Gen Prodrugs: Acyloxymethyl derivatives demonstrate superior in vivo efficacy, reducing HT-29 tumor volume by 60% compared to 30% for (R)-Tegafur in xenograft models .
Combination Therapies : S-1’s triple-component design reduces diarrhea incidence to 12% vs. 28% for UFT, validating its improved safety .
Preparation Methods
Preparation Method 1: Direct Catalytic Reaction with Tetrahydrofuran
Reaction Scheme and Conditions
This method involves reacting 5-fluorouracil with tetrahydrofuran in the presence of a catalyst and an oxidizing agent, typically copper acetate and hydrogen peroxide, in a polar aprotic solvent such as N,N-dimethylformamide (DMF).
Reagents and amounts (example scale):
- 5-fluorouracil: 13.00 g (0.10 mol)
- Tetrahydrofuran: 11.54 g (0.16 mol)
- Copper acetate: ~180–200 mg (0.9–1.0 mmol)
- Hydrogen peroxide (30% solution) or tert-butyl peroxide: ~22–28 g (0.25 mol)
- N,N-dimethylformamide: 100 mL
-
- Mix reagents and heat to 40 °C with stirring.
- Reaction time: 6–8 hours.
- Filter the reaction mixture.
- Extract the product by adding the filtrate to a mixture of ice water and trichloromethane.
- Wash the organic phase with purified water and saturated saline.
- Dry over anhydrous sodium sulfate.
- Concentrate under reduced pressure to obtain crude product.
- Recrystallize from anhydrous methanol to purify.
Yields and Purity
- Yield of refined product: approximately 88–90%
- Purity by HPLC: >99.8%
- Maximum single impurity: ~0.02–0.03%
- Retention time in HPLC: ~13.85 minutes
Advantages and Notes
- The use of copper acetate and peroxide allows for efficient catalysis.
- The reaction proceeds under mild heating (40 °C).
- Recrystallization ensures high purity.
- The method is scalable and has been patented with detailed process controls.
Preparation Method 2: Silylation and Reaction with 2-Chlorotetrahydrofuran
Reaction Scheme and Conditions
This method involves two main steps:
- Silylation of 5-fluorouracil to form bis-trimethylsilyl-5-fluorouracil using hexamethyldisilazane or chlorotrimethylsilane in the presence of triethylamine.
- Reaction of the silylated intermediate with 2-chlorotetrahydrofuran in dry methylene chloride at low temperature (-65 °C to solvent freezing point) in the presence of molecular sieves.
Detailed Procedure
-
- React 5-fluorouracil with hexamethyldisilazane at reflux.
- Remove excess reagents by volatilization.
-
- Under inert nitrogen atmosphere, add bis-trimethylsilyl-5-fluorouracil to a cold solution of 2-chlorotetrahydrofuran in methylene chloride containing 4 Å molecular sieves.
- Maintain temperature between -65 °C and the freezing point of the solvent.
- Stir to allow reaction and removal of water and HCl by molecular sieves.
-
- Slowly add the reaction mixture to a basic solution of ammonium hydroxide in methanol, maintaining pH above 7.5.
- Control temperature between -78 °C and -10 °C during addition to manage exothermicity.
- Warm to room temperature to precipitate the product.
- Filter, wash, and dry the precipitate.
- Adjust pH with dry ice (CO2) if necessary to optimize purity.
Yields and Purity
- Reported yields range from 50% to 65%, limited by side reactions involving hydrogen chloride by-product.
- Purity is high after purification steps.
- The method requires careful control of moisture and temperature.
Advantages and Challenges
- Provides an improved synthetic route with better control over reaction intermediates.
- Use of molecular sieves effectively removes water and HCl, improving product stability.
- Requires inert atmosphere and low-temperature conditions, increasing complexity.
- Side reactions due to HCl can reduce yield.
Comparative Data Table
Aspect | Direct Catalytic Reaction (Method 1) | Silylation + 2-Chlorotetrahydrofuran (Method 2) |
---|---|---|
Starting Materials | 5-fluorouracil, tetrahydrofuran | 5-fluorouracil, hexamethyldisilazane, 2-chlorotetrahydrofuran |
Catalyst/Oxidant | Copper acetate, hydrogen peroxide or tert-butyl peroxide | Triethylamine, molecular sieves |
Solvent | N,N-dimethylformamide | Dry methylene chloride |
Reaction Temperature | 40 °C | -65 °C to solvent freezing point |
Atmosphere | Ambient | Inert nitrogen atmosphere |
Reaction Time | 6–8 hours | Several hours with careful addition steps |
Yield | ~88–90% | 50–65% |
Purity (HPLC) | >99.8% | High after purification |
Main By-products/Issues | Minimal impurities | Hydrogen chloride causes bond cleavage, lowering yield |
Scalability | High | More complex due to low temperature and inert atmosphere |
Summary of Research Findings and Recommendations
- The direct catalytic reaction method is more straightforward, offers higher yields, and is suitable for industrial scale-up with relatively mild conditions.
- The silylation method , while more complex, provides an alternative synthetic route that can be advantageous when specific purity or reaction control is required.
- Both methods rely on careful purification steps such as recrystallization and pH control to achieve high purity.
- The choice of method depends on available equipment, desired scale, and purity requirements.
- Continuous improvements focus on minimizing by-products such as hydrogen chloride and optimizing reaction parameters to maximize yield.
Properties
IUPAC Name |
5-fluoro-1-[(2R)-oxolan-2-yl]pyrimidine-2,4-dione | |
---|---|---|
Source | PubChem | |
URL | https://pubchem.ncbi.nlm.nih.gov | |
Description | Data deposited in or computed by PubChem | |
InChI |
InChI=1S/C8H9FN2O3/c9-5-4-11(6-2-1-3-14-6)8(13)10-7(5)12/h4,6H,1-3H2,(H,10,12,13)/t6-/m1/s1 | |
Source | PubChem | |
URL | https://pubchem.ncbi.nlm.nih.gov | |
Description | Data deposited in or computed by PubChem | |
InChI Key |
WFWLQNSHRPWKFK-ZCFIWIBFSA-N | |
Source | PubChem | |
URL | https://pubchem.ncbi.nlm.nih.gov | |
Description | Data deposited in or computed by PubChem | |
Canonical SMILES |
C1CC(OC1)N2C=C(C(=O)NC2=O)F | |
Source | PubChem | |
URL | https://pubchem.ncbi.nlm.nih.gov | |
Description | Data deposited in or computed by PubChem | |
Isomeric SMILES |
C1C[C@@H](OC1)N2C=C(C(=O)NC2=O)F | |
Source | PubChem | |
URL | https://pubchem.ncbi.nlm.nih.gov | |
Description | Data deposited in or computed by PubChem | |
Molecular Formula |
C8H9FN2O3 | |
Source | PubChem | |
URL | https://pubchem.ncbi.nlm.nih.gov | |
Description | Data deposited in or computed by PubChem | |
DSSTOX Substance ID |
DTXSID8021305 | |
Record name | (R)-Tegafur | |
Source | EPA DSSTox | |
URL | https://comptox.epa.gov/dashboard/DTXSID8021305 | |
Description | DSSTox provides a high quality public chemistry resource for supporting improved predictive toxicology. | |
Molecular Weight |
200.17 g/mol | |
Source | PubChem | |
URL | https://pubchem.ncbi.nlm.nih.gov | |
Description | Data deposited in or computed by PubChem | |
CAS No. |
37076-68-9 | |
Record name | (R)-Ftorafur | |
Source | CAS Common Chemistry | |
URL | https://commonchemistry.cas.org/detail?cas_rn=37076-68-9 | |
Description | CAS Common Chemistry is an open community resource for accessing chemical information. Nearly 500,000 chemical substances from CAS REGISTRY cover areas of community interest, including common and frequently regulated chemicals, and those relevant to high school and undergraduate chemistry classes. This chemical information, curated by our expert scientists, is provided in alignment with our mission as a division of the American Chemical Society. | |
Explanation | The data from CAS Common Chemistry is provided under a CC-BY-NC 4.0 license, unless otherwise stated. | |
Record name | Tegafur, (R)- | |
Source | ChemIDplus | |
URL | https://pubchem.ncbi.nlm.nih.gov/substance/?source=chemidplus&sourceid=0037076689 | |
Description | ChemIDplus is a free, web search system that provides access to the structure and nomenclature authority files used for the identification of chemical substances cited in National Library of Medicine (NLM) databases, including the TOXNET system. | |
Record name | Fluorofur | |
Source | DTP/NCI | |
URL | https://dtp.cancer.gov/dtpstandard/servlet/dwindex?searchtype=NSC&outputformat=html&searchlist=200695 | |
Description | The NCI Development Therapeutics Program (DTP) provides services and resources to the academic and private-sector research communities worldwide to facilitate the discovery and development of new cancer therapeutic agents. | |
Explanation | Unless otherwise indicated, all text within NCI products is free of copyright and may be reused without our permission. Credit the National Cancer Institute as the source. | |
Record name | tegafur | |
Source | DTP/NCI | |
URL | https://dtp.cancer.gov/dtpstandard/servlet/dwindex?searchtype=NSC&outputformat=html&searchlist=148958 | |
Description | The NCI Development Therapeutics Program (DTP) provides services and resources to the academic and private-sector research communities worldwide to facilitate the discovery and development of new cancer therapeutic agents. | |
Explanation | Unless otherwise indicated, all text within NCI products is free of copyright and may be reused without our permission. Credit the National Cancer Institute as the source. | |
Record name | (R)-Tegafur | |
Source | EPA DSSTox | |
URL | https://comptox.epa.gov/dashboard/DTXSID8021305 | |
Description | DSSTox provides a high quality public chemistry resource for supporting improved predictive toxicology. | |
Record name | TEGAFUR, (R)- | |
Source | FDA Global Substance Registration System (GSRS) | |
URL | https://gsrs.ncats.nih.gov/ginas/app/beta/substances/5317337V7V | |
Description | The FDA Global Substance Registration System (GSRS) enables the efficient and accurate exchange of information on what substances are in regulated products. Instead of relying on names, which vary across regulatory domains, countries, and regions, the GSRS knowledge base makes it possible for substances to be defined by standardized, scientific descriptions. | |
Explanation | Unless otherwise noted, the contents of the FDA website (www.fda.gov), both text and graphics, are not copyrighted. They are in the public domain and may be republished, reprinted and otherwise used freely by anyone without the need to obtain permission from FDA. Credit to the U.S. Food and Drug Administration as the source is appreciated but not required. | |
Synthesis routes and methods I
Procedure details
Synthesis routes and methods II
Procedure details
Synthesis routes and methods III
Procedure details
Synthesis routes and methods IV
Procedure details
Synthesis routes and methods V
Procedure details
Retrosynthesis Analysis
AI-Powered Synthesis Planning: Our tool employs the Template_relevance Pistachio, Template_relevance Bkms_metabolic, Template_relevance Pistachio_ringbreaker, Template_relevance Reaxys, Template_relevance Reaxys_biocatalysis model, leveraging a vast database of chemical reactions to predict feasible synthetic routes.
One-Step Synthesis Focus: Specifically designed for one-step synthesis, it provides concise and direct routes for your target compounds, streamlining the synthesis process.
Accurate Predictions: Utilizing the extensive PISTACHIO, BKMS_METABOLIC, PISTACHIO_RINGBREAKER, REAXYS, REAXYS_BIOCATALYSIS database, our tool offers high-accuracy predictions, reflecting the latest in chemical research and data.
Strategy Settings
Precursor scoring | Relevance Heuristic |
---|---|
Min. plausibility | 0.01 |
Model | Template_relevance |
Template Set | Pistachio/Bkms_metabolic/Pistachio_ringbreaker/Reaxys/Reaxys_biocatalysis |
Top-N result to add to graph | 6 |
Feasible Synthetic Routes
Disclaimer and Information on In-Vitro Research Products
Please be aware that all articles and product information presented on BenchChem are intended solely for informational purposes. The products available for purchase on BenchChem are specifically designed for in-vitro studies, which are conducted outside of living organisms. In-vitro studies, derived from the Latin term "in glass," involve experiments performed in controlled laboratory settings using cells or tissues. It is important to note that these products are not categorized as medicines or drugs, and they have not received approval from the FDA for the prevention, treatment, or cure of any medical condition, ailment, or disease. We must emphasize that any form of bodily introduction of these products into humans or animals is strictly prohibited by law. It is essential to adhere to these guidelines to ensure compliance with legal and ethical standards in research and experimentation.