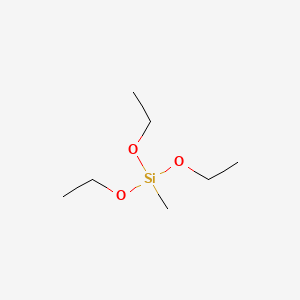
Triethoxymethylsilane
Overview
Description
Triethoxymethylsilane, also known as Methyltriethoxysilane (MTES), is an organosilane compound . It has a chemical formula of (C₂H₅O)₃SiCH₃ and a molar mass of 178.3 g/mol . It is a colorless, free-flowing liquid .
Synthesis Analysis
The selective synthesis of triethoxysilane can be achieved through the direct reaction between ethanol and silicon, catalyzed by CuCl and Cu0 nano .Molecular Structure Analysis
The molecular structure of this compound is represented by the formula (C₂H₅O)₃SiCH₃ . It has a Hill Formula of C₇H₁₈O₃Si .Chemical Reactions Analysis
This compound is used in precious metal-catalysed hydrosilylation reactions . The resulting triethoxysilyl groups are often valued for attachment to silica surfaces .Physical And Chemical Properties Analysis
This compound has a boiling point of 142 °C (1013 hPa), a density of 0.89 g/cm3 (20 °C), a flash point of 34 °C, an ignition temperature of 220 °C, and a vapor pressure of 15 hPa (20 °C) . It has a melting point of <-40 °C .Scientific Research Applications
Surface Modification of Ceramic Membranes : Hendren, Brant, and Wiesner (2009) explored surface treatments to make ceramic membranes hydrophobic, suitable for use in direct contact membrane distillation (DCMD). They assessed treatments like perfluorodecyltriethoxysilane and trichloromethylsilane. The perfluorodecyltriethoxysilane-treated membrane showed superior performance due to its optimized pore structure (Hendren, Brant, & Wiesner, 2009).
Organosilicon Compound Fragmentation Study : Tabei et al. (1993) investigated the fragmentation of triethoxymethylsilane by electron impact, revealing complex molecular behaviors. This study provides insights into the chemical behavior of organosilicon compounds, useful in various fields like materials science (Tabei et al., 1993).
Biomedical Applications : Beele et al. (1992) researched the biocompatibility of polyoxyethylene network films made from triethoxysilane-terminated prepolymers, testing them on human and chicken skin cultures. This study highlights the potential of this compound derivatives in biomedical applications (Beele et al., 1992).
Elastomeric Networks : Sur and Mark (1985) demonstrated the use of triethoxysilyl-terminated poly(dimethylsiloxane) for creating stable elastomeric networks with silica or titania fillers. This has implications for material science, especially in developing novel elastomers (Sur & Mark, 1985).
Gene Delivery System Development : Zhang et al. (2013) explored (3-aminopropyl)triethoxysilane-modified iron oxide nanoparticles for biomedical applications, including gene delivery. They developed a system that improved gene-binding capacity and transfection efficiency, indicating potential applications in gene therapy (Zhang et al., 2013).
Microreactor Development for Chemical Reactions : Wan et al. (2004) used silicon-glass microreactors with TS-1 catalyst wall coatings, modified with this compound, for 1-pentene epoxidation. This study is significant for chemical engineering and process technology (Wan et al., 2004).
Mechanism of Action
Target of Action
Methyltriethoxysilane, also known as Triethoxymethylsilane, is primarily targeted at inorganic materials such as cellulose . It is used as a coupling agent to modify the surface of these materials, enhancing their properties for various applications .
Mode of Action
Methyltriethoxysilane interacts with its targets through a process known as silylation . This involves the formation of covalent bonds between the silicon atom in Methyltriethoxysilane and the hydroxyl groups present on the surface of the target material . The result is a modification of the surface properties of the material, such as its hydrophobicity .
Biochemical Pathways
The primary biochemical pathway affected by Methyltriethoxysilane is the silylation process . This process involves the hydrolysis of Methyltriethoxysilane to form different hydrolysis products, which then react with the target material . The hydrolysis product with two hydroxyl groups is more favorable for the growth of organosilicon on the cellulose surface .
Result of Action
The action of Methyltriethoxysilane results in the modification of the surface properties of the target material . For instance, when used on cellulose, it improves the hydrophobicity of the cellulose surface . This can enhance the material’s resistance to water, making it more suitable for various applications .
Action Environment
The action of Methyltriethoxysilane can be influenced by various environmental factors. For example, the rate of hydrolysis and subsequent silylation can be affected by factors such as temperature and the presence of moisture . Additionally, the stability and efficacy of Methyltriethoxysilane can be influenced by storage conditions, including temperature and exposure to light .
Safety and Hazards
Triethoxymethylsilane is classified as a flammable liquid and vapor . Precautionary measures include keeping away from heat, hot surfaces, sparks, open flames, and other ignition sources . The container should be kept tightly closed, and the container and receiving equipment should be grounded and bonded .
Future Directions
Biochemical Analysis
Biochemical Properties
Methyltriethoxysilane is a hydrophobic silica precursor because it has a methyl group that functions as a hydrophobicity guiding agent . It is used in the production of silicone polymers or silicone resin
Cellular Effects
It has been shown that the addition of Methyltriethoxysilane can improve the waterproof property of certain composites . This suggests that it may have an impact on cell function, potentially influencing cell signaling pathways, gene expression, and cellular metabolism.
Molecular Mechanism
It is known that Methyltriethoxysilane is usually prepared from methyltrichlorosilane and methanol
Temporal Effects in Laboratory Settings
It is known that Methyltriethoxysilane hydrolyzes slowly in the presence of moisture to form reactive silanols . These react further to produce oligosiloxanes and then polysiloxanes .
Metabolic Pathways
It is known that Methyltriethoxysilane is a hydrophobic silica precursor, suggesting that it may be involved in the metabolism of silicon-based compounds .
Transport and Distribution
It is known that Methyltriethoxysilane is a hydrophobic silica precursor, suggesting that it may interact with hydrophobic regions within cells and tissues .
Subcellular Localization
Given its hydrophobic nature, it may localize to hydrophobic regions within the cell .
properties
IUPAC Name |
triethoxy(methyl)silane | |
---|---|---|
Source | PubChem | |
URL | https://pubchem.ncbi.nlm.nih.gov | |
Description | Data deposited in or computed by PubChem | |
InChI |
InChI=1S/C7H18O3Si/c1-5-8-11(4,9-6-2)10-7-3/h5-7H2,1-4H3 | |
Source | PubChem | |
URL | https://pubchem.ncbi.nlm.nih.gov | |
Description | Data deposited in or computed by PubChem | |
InChI Key |
CPUDPFPXCZDNGI-UHFFFAOYSA-N | |
Source | PubChem | |
URL | https://pubchem.ncbi.nlm.nih.gov | |
Description | Data deposited in or computed by PubChem | |
Canonical SMILES |
CCO[Si](C)(OCC)OCC | |
Source | PubChem | |
URL | https://pubchem.ncbi.nlm.nih.gov | |
Description | Data deposited in or computed by PubChem | |
Molecular Formula |
C7H18O3Si | |
Source | PubChem | |
URL | https://pubchem.ncbi.nlm.nih.gov | |
Description | Data deposited in or computed by PubChem | |
Related CAS |
25930-91-0 | |
Record name | Methyltriethoxysilane homopolymer | |
Source | CAS Common Chemistry | |
URL | https://commonchemistry.cas.org/detail?cas_rn=25930-91-0 | |
Description | CAS Common Chemistry is an open community resource for accessing chemical information. Nearly 500,000 chemical substances from CAS REGISTRY cover areas of community interest, including common and frequently regulated chemicals, and those relevant to high school and undergraduate chemistry classes. This chemical information, curated by our expert scientists, is provided in alignment with our mission as a division of the American Chemical Society. | |
Explanation | The data from CAS Common Chemistry is provided under a CC-BY-NC 4.0 license, unless otherwise stated. | |
DSSTOX Substance ID |
DTXSID6042490 | |
Record name | Triethoxymethylsilane | |
Source | EPA DSSTox | |
URL | https://comptox.epa.gov/dashboard/DTXSID6042490 | |
Description | DSSTox provides a high quality public chemistry resource for supporting improved predictive toxicology. | |
Molecular Weight |
178.30 g/mol | |
Source | PubChem | |
URL | https://pubchem.ncbi.nlm.nih.gov | |
Description | Data deposited in or computed by PubChem | |
Physical Description |
Liquid, Clear colorless liquid; [Acros Organics MSDS] | |
Record name | Silane, triethoxymethyl- | |
Source | EPA Chemicals under the TSCA | |
URL | https://www.epa.gov/chemicals-under-tsca | |
Description | EPA Chemicals under the Toxic Substances Control Act (TSCA) collection contains information on chemicals and their regulations under TSCA, including non-confidential content from the TSCA Chemical Substance Inventory and Chemical Data Reporting. | |
Record name | Methyltriethoxysilane | |
Source | Haz-Map, Information on Hazardous Chemicals and Occupational Diseases | |
URL | https://haz-map.com/Agents/15631 | |
Description | Haz-Map® is an occupational health database designed for health and safety professionals and for consumers seeking information about the adverse effects of workplace exposures to chemical and biological agents. | |
Explanation | Copyright (c) 2022 Haz-Map(R). All rights reserved. Unless otherwise indicated, all materials from Haz-Map are copyrighted by Haz-Map(R). No part of these materials, either text or image may be used for any purpose other than for personal use. Therefore, reproduction, modification, storage in a retrieval system or retransmission, in any form or by any means, electronic, mechanical or otherwise, for reasons other than personal use, is strictly prohibited without prior written permission. | |
Vapor Pressure |
11.0 [mmHg] | |
Record name | Methyltriethoxysilane | |
Source | Haz-Map, Information on Hazardous Chemicals and Occupational Diseases | |
URL | https://haz-map.com/Agents/15631 | |
Description | Haz-Map® is an occupational health database designed for health and safety professionals and for consumers seeking information about the adverse effects of workplace exposures to chemical and biological agents. | |
Explanation | Copyright (c) 2022 Haz-Map(R). All rights reserved. Unless otherwise indicated, all materials from Haz-Map are copyrighted by Haz-Map(R). No part of these materials, either text or image may be used for any purpose other than for personal use. Therefore, reproduction, modification, storage in a retrieval system or retransmission, in any form or by any means, electronic, mechanical or otherwise, for reasons other than personal use, is strictly prohibited without prior written permission. | |
CAS RN |
2031-67-6 | |
Record name | Triethoxymethylsilane | |
Source | CAS Common Chemistry | |
URL | https://commonchemistry.cas.org/detail?cas_rn=2031-67-6 | |
Description | CAS Common Chemistry is an open community resource for accessing chemical information. Nearly 500,000 chemical substances from CAS REGISTRY cover areas of community interest, including common and frequently regulated chemicals, and those relevant to high school and undergraduate chemistry classes. This chemical information, curated by our expert scientists, is provided in alignment with our mission as a division of the American Chemical Society. | |
Explanation | The data from CAS Common Chemistry is provided under a CC-BY-NC 4.0 license, unless otherwise stated. | |
Record name | Triethoxymethylsilane | |
Source | ChemIDplus | |
URL | https://pubchem.ncbi.nlm.nih.gov/substance/?source=chemidplus&sourceid=0002031676 | |
Description | ChemIDplus is a free, web search system that provides access to the structure and nomenclature authority files used for the identification of chemical substances cited in National Library of Medicine (NLM) databases, including the TOXNET system. | |
Record name | Methyltriethoxysilane | |
Source | DTP/NCI | |
URL | https://dtp.cancer.gov/dtpstandard/servlet/dwindex?searchtype=NSC&outputformat=html&searchlist=5226 | |
Description | The NCI Development Therapeutics Program (DTP) provides services and resources to the academic and private-sector research communities worldwide to facilitate the discovery and development of new cancer therapeutic agents. | |
Explanation | Unless otherwise indicated, all text within NCI products is free of copyright and may be reused without our permission. Credit the National Cancer Institute as the source. | |
Record name | Silane, triethoxymethyl- | |
Source | EPA Chemicals under the TSCA | |
URL | https://www.epa.gov/chemicals-under-tsca | |
Description | EPA Chemicals under the Toxic Substances Control Act (TSCA) collection contains information on chemicals and their regulations under TSCA, including non-confidential content from the TSCA Chemical Substance Inventory and Chemical Data Reporting. | |
Record name | Triethoxymethylsilane | |
Source | EPA DSSTox | |
URL | https://comptox.epa.gov/dashboard/DTXSID6042490 | |
Description | DSSTox provides a high quality public chemistry resource for supporting improved predictive toxicology. | |
Record name | Triethoxy(methyl)silane | |
Source | European Chemicals Agency (ECHA) | |
URL | https://echa.europa.eu/substance-information/-/substanceinfo/100.016.350 | |
Description | The European Chemicals Agency (ECHA) is an agency of the European Union which is the driving force among regulatory authorities in implementing the EU's groundbreaking chemicals legislation for the benefit of human health and the environment as well as for innovation and competitiveness. | |
Explanation | Use of the information, documents and data from the ECHA website is subject to the terms and conditions of this Legal Notice, and subject to other binding limitations provided for under applicable law, the information, documents and data made available on the ECHA website may be reproduced, distributed and/or used, totally or in part, for non-commercial purposes provided that ECHA is acknowledged as the source: "Source: European Chemicals Agency, http://echa.europa.eu/". Such acknowledgement must be included in each copy of the material. ECHA permits and encourages organisations and individuals to create links to the ECHA website under the following cumulative conditions: Links can only be made to webpages that provide a link to the Legal Notice page. | |
Record name | TRIETHOXYMETHYLSILANE | |
Source | FDA Global Substance Registration System (GSRS) | |
URL | https://gsrs.ncats.nih.gov/ginas/app/beta/substances/N811L6SAVL | |
Description | The FDA Global Substance Registration System (GSRS) enables the efficient and accurate exchange of information on what substances are in regulated products. Instead of relying on names, which vary across regulatory domains, countries, and regions, the GSRS knowledge base makes it possible for substances to be defined by standardized, scientific descriptions. | |
Explanation | Unless otherwise noted, the contents of the FDA website (www.fda.gov), both text and graphics, are not copyrighted. They are in the public domain and may be republished, reprinted and otherwise used freely by anyone without the need to obtain permission from FDA. Credit to the U.S. Food and Drug Administration as the source is appreciated but not required. | |
Synthesis routes and methods
Procedure details
Retrosynthesis Analysis
AI-Powered Synthesis Planning: Our tool employs the Template_relevance Pistachio, Template_relevance Bkms_metabolic, Template_relevance Pistachio_ringbreaker, Template_relevance Reaxys, Template_relevance Reaxys_biocatalysis model, leveraging a vast database of chemical reactions to predict feasible synthetic routes.
One-Step Synthesis Focus: Specifically designed for one-step synthesis, it provides concise and direct routes for your target compounds, streamlining the synthesis process.
Accurate Predictions: Utilizing the extensive PISTACHIO, BKMS_METABOLIC, PISTACHIO_RINGBREAKER, REAXYS, REAXYS_BIOCATALYSIS database, our tool offers high-accuracy predictions, reflecting the latest in chemical research and data.
Strategy Settings
Precursor scoring | Relevance Heuristic |
---|---|
Min. plausibility | 0.01 |
Model | Template_relevance |
Template Set | Pistachio/Bkms_metabolic/Pistachio_ringbreaker/Reaxys/Reaxys_biocatalysis |
Top-N result to add to graph | 6 |
Feasible Synthetic Routes
Disclaimer and Information on In-Vitro Research Products
Please be aware that all articles and product information presented on BenchChem are intended solely for informational purposes. The products available for purchase on BenchChem are specifically designed for in-vitro studies, which are conducted outside of living organisms. In-vitro studies, derived from the Latin term "in glass," involve experiments performed in controlled laboratory settings using cells or tissues. It is important to note that these products are not categorized as medicines or drugs, and they have not received approval from the FDA for the prevention, treatment, or cure of any medical condition, ailment, or disease. We must emphasize that any form of bodily introduction of these products into humans or animals is strictly prohibited by law. It is essential to adhere to these guidelines to ensure compliance with legal and ethical standards in research and experimentation.