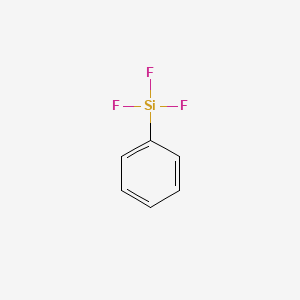
Phenyltrifluorosilane
Overview
Description
Phenyltrifluorosilane (C₆H₅SiF₃) is an organosilicon compound characterized by a silicon atom bonded to a phenyl group and three fluorine atoms. Its unique electronic structure, particularly the electron-withdrawing nature of the -SiF₃ group, enables it to act as a strong tetrel bond (TtB) donor. Tetrel bonds, a type of σ-hole interaction, involve the electrophilic silicon center attracting electron-rich species like oxygen or nitrogen . This compound is widely studied in supramolecular chemistry and catalysis due to its ability to form stable complexes with Lewis bases such as dimethyl sulfoxide (DMSO) .
Preparation Methods
Synthetic Routes and Reaction Conditions: Phenyltrifluorosilane can be synthesized through several methods. One common approach involves the reaction of phenylsilane with a fluorinating agent such as hydrogen fluoride or a fluorine-containing compound under controlled conditions. The reaction typically proceeds as follows: [ \text{PhSiH}_3 + 3 \text{HF} \rightarrow \text{PhSiF}_3 + 3 \text{H}_2 ]
Industrial Production Methods: Industrial production of this compound often involves the use of large-scale reactors where phenylsilane is reacted with fluorine gas or hydrogen fluoride. The process is carefully controlled to ensure high yield and purity of the final product .
Chemical Reactions Analysis
Chemical Reactions of Phenyltrifluorosilane
This compound undergoes several significant chemical reactions, which can be categorized into halogenation, hydrolysis, and photochemical dissociation.
Halogenation Reactions
This compound can participate in halogenation reactions where it acts as a precursor to produce various halogenated silanes. The following reactions illustrate this process:
-
Reaction with Hydroiodic Acid (HI) :
This reaction demonstrates the substitution of one fluorine atom with iodine, facilitated by aluminum triiodide as a Lewis acid catalyst. Yields can vary based on reaction conditions, with reported yields of up to 80% for iodo-trifluorosilane under optimized conditions .
-
Reaction with Bromine :
Under photochemical conditions, this compound can react with bromine (Br) leading to the formation of brominated derivatives. The main products are typically brominated silanes alongside the elimination of SiF and hydrogen:
Hydrolysis
Upon contact with water, this compound hydrolyzes to form phenylsiloxanes and hydrofluoric acid (HF). The general reaction can be represented as:
This reaction highlights the reactivity of the fluorine atoms, which are replaced by hydroxyl groups during hydrolysis.
Photochemical Reactions
This compound also exhibits interesting behavior under infrared multiphoton dissociation (IR MPD) conditions. Studies have shown that upon exposure to infrared radiation, it can dissociate into various products, including silicon isotopes and fluorinated species:
-
The main dissociation channels include:
-
Elimination of SiF
-
Elimination of hydrogen
-
This photochemical behavior is significant for applications in isotope separation and material synthesis .
Data Tables
Scientific Research Applications
Silicon-Based Polymers
Phenyltrifluorosilane as a Precursor:
PTS is utilized as a precursor in the synthesis of silicon-based polymers. These polymers exhibit enhanced thermal stability and mechanical properties due to the incorporation of fluorinated groups, which improve their resistance to degradation under harsh conditions .
Applications:
- Coatings: PTS-derived polymers are employed in protective coatings that require durability and chemical resistance.
- Sealants and Adhesives: The unique properties of PTS contribute to the formulation of sealants and adhesives that perform well in extreme environments.
Supramolecular Chemistry
Tetrel Bonding:
this compound participates in tetrel bonding interactions, which are significant in supramolecular chemistry. Tetrel bonds involve a σ-hole on the silicon atom that can interact with Lewis bases, facilitating the formation of stable complexes .
Research Findings:
- Studies have shown that PTS can form strong tetrel bonds with various electron donor molecules, leading to new insights into molecular recognition processes and crystal engineering .
- The interaction energies of these complexes can reach up to 70 kcal/mol, indicating strong bonding capabilities .
Organic Synthesis
Reactivity in Chemical Reactions:
PTS is involved in several chemical reactions that lead to the formation of new heterocyclic compounds. For example, its reaction with 8-hydroxyquinoline results in the cleavage of the C–Si bond, allowing for the transfer of the SiF group and leading to pentacoordinate silicon compounds .
Applications:
- Synthesis of Fluorinated Compounds: PTS serves as a fluorinating agent, introducing trifluoromethyl groups into organic molecules, which can modify their biological activity and reactivity.
- Drug Development: The incorporation of fluorinated groups is crucial in designing pharmaceuticals with improved efficacy and selectivity.
Material Science
Advanced Materials:
The unique properties of PTS make it suitable for developing advanced materials with specific functionalities. Its fluorinated nature contributes to low surface energy and hydrophobic characteristics, making it ideal for applications in:
- Self-cleaning surfaces: Utilizing PTS-derived materials can lead to surfaces that repel water and dirt.
- Optoelectronic devices: Fluorinated silicon compounds are explored for use in electronic devices due to their favorable electronic properties.
Case Studies
Mechanism of Action
The mechanism by which phenyltrifluorosilane exerts its effects involves the formation of tetrel bonds with Lewis bases. The silicon atom in this compound has an empty p orbital that can accept electron density from the lone pairs of Lewis bases, leading to the formation of a stable complex. This interaction is primarily electrostatic in nature but also involves significant charge transfer .
Comparison with Similar Compounds
Structural and Electronic Properties
Table 1: Key Parameters of Phenyltrifluorosilane and Analogous Compounds
*Estimated based on analogous tetrel bond studies.
- This compound vs. Phenyltrichlorosilane : The -SiF₃ group in this compound creates a stronger σ-hole than -SiCl₃ due to fluorine’s higher electronegativity, leading to shorter Si···O distances (-SO₃H substituent: 2.02 Å vs. ~2.8 Å in chlorinated analogs) and higher interaction energies (up to -122.51 kJ/mol vs. -50 kJ/mol) .
- This compound vs. CF₃X : Unlike CF₃X (X = Cl, Br), which primarily forms halogen bonds, this compound preferentially engages in tetrel bonding. For example, SiF₃X complexes with DMSO exhibit stronger interactions (-122.51 kJ/mol) compared to halogen-bonded CF₃X-DMSO systems (-90 kJ/mol) .
Substituent Effects
Electron-withdrawing substituents (e.g., -SO₃H, -CN) on the phenyl ring enhance the σ-hole magnitude, increasing tetrel bond strength. Conversely, electron-donating groups (e.g., -NH₂, -OCH₃) weaken the interaction. For instance:
- -SO₃H substituent : Interaction energy = -122.51 kJ/mol, Si···O distance = 2.02 Å .
- -NH₂ substituent : Interaction energy = -16.29 kJ/mol, Si···O distance = 3.00 Å .
This trend correlates linearly with Hammett constants (σₚ), confirming the electronic tunability of tetrel bonds .
Competition with Other Interactions
This compound’s tetrel bonds compete with hydrogen and halogen bonds in multicomponent systems:
- Hydrogen bonds : In DMSO complexes with -NH₂ or -OH substituents, hydrogen bonding dominates over tetrel bonding due to stronger electrostatic contributions .
- Halogen bonds : CF₃X forms halogen bonds with DMSO, but SiF₃X favors tetrel bonds even in the presence of halogen substituents .
Basis Set Sensitivity
The choice of computational basis sets significantly impacts results. For this compound-DMSO complexes:
- Smaller basis sets (e.g., aug-cc-pVDZ) overestimate interaction energies (e.g., ΔE = -112.42 kJ/mol for -CH₃ substituent) due to inadequate electron correlation .
- The aug’-cc-pVTZ basis set provides reliable geometries and energies, capturing partial covalency in strong tetrel bonds (e.g., -SO₃H systems) .
Biological Activity
Phenyltrifluorosilane (PTS) is an organosilicon compound that has garnered interest due to its unique chemical properties and potential biological activities. This article explores the biological activity of PTS, focusing on its interactions, reactions, and implications in medicinal chemistry.
This compound has the molecular formula C₆H₅SiF₃. Its structure includes a phenyl group attached to a silicon atom that is further bonded to three fluorine atoms. The presence of the trifluorosilane group imparts distinct reactivity, particularly in cross-coupling reactions and interactions with various biological molecules.
1. Tetrel Bonding
PTS exhibits significant tetrel bonding capabilities, which are interactions between a silicon atom and electronegative atoms such as oxygen or nitrogen. Research indicates that PTS can form stable complexes with molecules like dimethyl sulfoxide (DMSO) through these interactions. The strength of these bonds can be influenced by substituents on the phenyl ring, affecting the biological activity of the resulting complexes .
2. Reactivity in Medicinal Chemistry
PTS has been utilized in various palladium-catalyzed cross-coupling reactions, which are crucial for synthesizing biologically active compounds. For instance, it has been involved in Hiyama-type cross-coupling reactions that facilitate the formation of carbon-carbon bonds, leading to the creation of compounds with potential antiviral and antifungal activities .
Case Study 1: Protodesilylation Reaction
A study investigated the protodesilylation reaction of PTS with 8-hydroxyquinoline. This reaction resulted in C-Si bond cleavage, leading to new heterocyclic compounds that exhibited enhanced biological properties. The cleavage of the Si-F bond was particularly notable, as it could lead to the formation of biologically relevant intermediates .
Case Study 2: Antimicrobial Activity
Another research effort highlighted the antimicrobial properties of compounds synthesized using PTS as a precursor. The derivatives formed through cross-coupling reactions demonstrated significant antibacterial activity against various pathogens, indicating PTS's utility in developing new antimicrobial agents .
Data Tables
Compound | Biological Activity | Reference |
---|---|---|
This compound | Antimicrobial | |
PTS Derivatives | Antiviral | |
Heterocyclic Compounds | Antitumor | |
PTS-DMSO Complex | Enhanced binding interactions |
Research Findings
- Antimicrobial Properties : Compounds derived from PTS exhibit broad-spectrum antimicrobial activity, making them candidates for further development in pharmaceuticals.
- Cross-Coupling Efficiency : The use of PTS in palladium-catalyzed reactions has shown to yield high product diversity and efficiency, with yields reaching up to 98% in certain applications .
- Biocompatibility : While PTS itself poses some toxicity concerns due to its fluorinated nature, research indicates that its derivatives can be designed to minimize adverse effects while retaining biological activity .
Q & A
Basic Research Questions
Q. What are the safety protocols for handling phenyltrifluorosilane in laboratory settings?
this compound requires stringent safety measures due to its reactivity and potential hazards. Key precautions include:
- Use of personal protective equipment (PPE): Nitrile gloves, lab coats, and chemical splash goggles .
- Avoid inhalation of vapors by working in a fume hood with proper ventilation .
- Immediate rinsing with water for 15 minutes in case of eye or skin contact, followed by medical evaluation .
- Storage in a cool, dry place away from oxidizing agents and moisture to prevent hazardous reactions (e.g., hydrolysis) .
Q. What are the standard synthetic routes for preparing this compound?
this compound is typically synthesized via:
- Direct fluorination : Reaction of phenylsilane (C₆H₅SiH₃) with fluorine gas under controlled conditions .
- Transmetalation : Exchange reactions using fluorinating agents like ZnF₂ or KF in anhydrous solvents (e.g., THF) .
- Purification : Distillation under inert atmosphere (argon/nitrogen) to isolate the product from byproducts such as polysilanes .
Q. How does this compound function in cross-coupling reactions?
this compound acts as a nucleophilic coupling partner in transition metal-catalyzed reactions. Key mechanisms include:
- Fluorine activation : The electron-withdrawing effect of fluorine ligands enhances silicon’s electrophilicity, facilitating transmetalation with palladium or nickel catalysts .
- Reaction optimization : Yields improve with promoters like ZnF₂ (65–85% yield in aryl-aryl couplings) and polar aprotic solvents (e.g., DMF) .
Advanced Research Questions
Q. How can enantioselectivity be controlled in this compound-mediated 1,4-additions to enones?
Enantioselective 1,4-additions require:
- Chiral ligands : Bidentate phosphine ligands (e.g., (R)-BINAP) paired with Pd(0) catalysts to achieve enantiomeric ratios (e.r.) up to 95:5 .
- Substrate tuning : Electron-deficient enones (e.g., β-nitrostyrene) enhance stereochemical control.
- Kinetic resolution : Monitoring reaction progress via chiral HPLC or NMR to identify optimal timepoints for product isolation .
Q. What computational methods validate the gas-phase thermochemistry of this compound?
Gas-phase studies employ:
- Mass spectrometry : Collision-induced dissociation (CID) to measure bond dissociation energies (BDEs) of Si–F and Si–C bonds .
- Density Functional Theory (DFT) : Benchmarking experimental ΔHf values (e.g., 227 kJ/mol for Si–F bond cleavage) against calculated Gibbs free energy profiles .
- Ion cyclotron resonance (ICR) : Quantifying proton affinity and ionization potentials for reaction pathway modeling .
Q. How can contradictions in reaction yields across catalytic systems be resolved?
Discrepancies often arise from:
- Catalyst poisoning : Trace moisture or oxygen degrades Pd(0) catalysts, reducing yields. Use of rigorously dried solvents and Schlenk techniques mitigates this .
- Ligand effects : Bulky ligands (e.g., P(t-Bu)₃) improve stability but may slow transmetalation. Screening ligand libraries identifies optimal balance .
- Solvent polarity : High-polarity solvents (e.g., DMSO) accelerate silane activation but may promote side reactions. Solvent optimization via Design of Experiments (DoE) is recommended .
Q. Methodological Considerations
- Data validation : Cross-reference experimental results with computational models (e.g., Gaussian09 for DFT) to confirm mechanistic hypotheses .
- Contradiction analysis : Use control experiments (e.g., isotopic labeling, kinetic isotope effects) to isolate variables affecting yield and selectivity .
- Safety compliance : Regularly update risk assessments using SDS sections 7 (handling) and 10 (incompatibilities) to prevent accidents .
Properties
IUPAC Name |
trifluoro(phenyl)silane | |
---|---|---|
Source | PubChem | |
URL | https://pubchem.ncbi.nlm.nih.gov | |
Description | Data deposited in or computed by PubChem | |
InChI |
InChI=1S/C6H5F3Si/c7-10(8,9)6-4-2-1-3-5-6/h1-5H | |
Source | PubChem | |
URL | https://pubchem.ncbi.nlm.nih.gov | |
Description | Data deposited in or computed by PubChem | |
InChI Key |
KGWNTHHPMKEAIK-UHFFFAOYSA-N | |
Source | PubChem | |
URL | https://pubchem.ncbi.nlm.nih.gov | |
Description | Data deposited in or computed by PubChem | |
Canonical SMILES |
C1=CC=C(C=C1)[Si](F)(F)F | |
Source | PubChem | |
URL | https://pubchem.ncbi.nlm.nih.gov | |
Description | Data deposited in or computed by PubChem | |
Molecular Formula |
C6H5F3Si | |
Source | PubChem | |
URL | https://pubchem.ncbi.nlm.nih.gov | |
Description | Data deposited in or computed by PubChem | |
DSSTOX Substance ID |
DTXSID90190267 | |
Record name | Silane, phenyltrifluoro- | |
Source | EPA DSSTox | |
URL | https://comptox.epa.gov/dashboard/DTXSID90190267 | |
Description | DSSTox provides a high quality public chemistry resource for supporting improved predictive toxicology. | |
Molecular Weight |
162.18 g/mol | |
Source | PubChem | |
URL | https://pubchem.ncbi.nlm.nih.gov | |
Description | Data deposited in or computed by PubChem | |
CAS No. |
368-47-8 | |
Record name | (Trifluorosilyl)benzene | |
Source | CAS Common Chemistry | |
URL | https://commonchemistry.cas.org/detail?cas_rn=368-47-8 | |
Description | CAS Common Chemistry is an open community resource for accessing chemical information. Nearly 500,000 chemical substances from CAS REGISTRY cover areas of community interest, including common and frequently regulated chemicals, and those relevant to high school and undergraduate chemistry classes. This chemical information, curated by our expert scientists, is provided in alignment with our mission as a division of the American Chemical Society. | |
Explanation | The data from CAS Common Chemistry is provided under a CC-BY-NC 4.0 license, unless otherwise stated. | |
Record name | Phenyltrifluorosilane | |
Source | ChemIDplus | |
URL | https://pubchem.ncbi.nlm.nih.gov/substance/?source=chemidplus&sourceid=0000368478 | |
Description | ChemIDplus is a free, web search system that provides access to the structure and nomenclature authority files used for the identification of chemical substances cited in National Library of Medicine (NLM) databases, including the TOXNET system. | |
Record name | Silane, phenyltrifluoro- | |
Source | EPA DSSTox | |
URL | https://comptox.epa.gov/dashboard/DTXSID90190267 | |
Description | DSSTox provides a high quality public chemistry resource for supporting improved predictive toxicology. | |
Record name | PHENYLTRIFLUOROSILANE | |
Source | FDA Global Substance Registration System (GSRS) | |
URL | https://gsrs.ncats.nih.gov/ginas/app/beta/substances/G9KX9P6ZV4 | |
Description | The FDA Global Substance Registration System (GSRS) enables the efficient and accurate exchange of information on what substances are in regulated products. Instead of relying on names, which vary across regulatory domains, countries, and regions, the GSRS knowledge base makes it possible for substances to be defined by standardized, scientific descriptions. | |
Explanation | Unless otherwise noted, the contents of the FDA website (www.fda.gov), both text and graphics, are not copyrighted. They are in the public domain and may be republished, reprinted and otherwise used freely by anyone without the need to obtain permission from FDA. Credit to the U.S. Food and Drug Administration as the source is appreciated but not required. | |
Retrosynthesis Analysis
AI-Powered Synthesis Planning: Our tool employs the Template_relevance Pistachio, Template_relevance Bkms_metabolic, Template_relevance Pistachio_ringbreaker, Template_relevance Reaxys, Template_relevance Reaxys_biocatalysis model, leveraging a vast database of chemical reactions to predict feasible synthetic routes.
One-Step Synthesis Focus: Specifically designed for one-step synthesis, it provides concise and direct routes for your target compounds, streamlining the synthesis process.
Accurate Predictions: Utilizing the extensive PISTACHIO, BKMS_METABOLIC, PISTACHIO_RINGBREAKER, REAXYS, REAXYS_BIOCATALYSIS database, our tool offers high-accuracy predictions, reflecting the latest in chemical research and data.
Strategy Settings
Precursor scoring | Relevance Heuristic |
---|---|
Min. plausibility | 0.01 |
Model | Template_relevance |
Template Set | Pistachio/Bkms_metabolic/Pistachio_ringbreaker/Reaxys/Reaxys_biocatalysis |
Top-N result to add to graph | 6 |
Feasible Synthetic Routes
Disclaimer and Information on In-Vitro Research Products
Please be aware that all articles and product information presented on BenchChem are intended solely for informational purposes. The products available for purchase on BenchChem are specifically designed for in-vitro studies, which are conducted outside of living organisms. In-vitro studies, derived from the Latin term "in glass," involve experiments performed in controlled laboratory settings using cells or tissues. It is important to note that these products are not categorized as medicines or drugs, and they have not received approval from the FDA for the prevention, treatment, or cure of any medical condition, ailment, or disease. We must emphasize that any form of bodily introduction of these products into humans or animals is strictly prohibited by law. It is essential to adhere to these guidelines to ensure compliance with legal and ethical standards in research and experimentation.