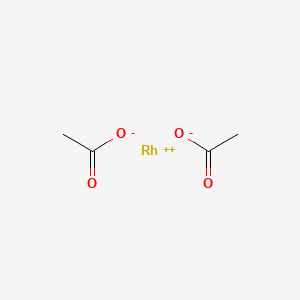
Rhodium(II) acetate
Overview
Description
Rhodium(II) acetate is a coordination compound with the formula Rh₂(CH₃CO₂)₄. This emerald green powder is slightly soluble in polar solvents, including water. It is widely studied as a transition metal carboxylate complex and is used as a catalyst for various organic transformations, such as cyclopropanation of alkenes .
Preparation Methods
Rhodium(II) acetate is typically prepared by heating hydrated rhodium(III) chloride in a methanol-acetic acid mixture. The crude product is the bis(methanol) complex, which is easily desolvated . Industrial production methods often involve similar processes, ensuring the compound’s purity and stability for catalytic applications .
Chemical Reactions Analysis
Rhodium(II) acetate undergoes various types of reactions, including:
Oxidation: It can catalyze the oxidation of alcohols.
Reduction: It is involved in hydrogenation reactions.
Substitution: The acetate group can be replaced by other carboxylates of strong acids.
Cyclopropanation: It catalyzes the cyclopropanation of alkenes
Insertion: It facilitates insertion into C-H and X-H bonds (X = N/S/O).
Common reagents and conditions used in these reactions include diazo compounds for cyclopropanation and alcohols for oxidation . Major products formed from these reactions are cyclopropanes, oxidized alcohols, and substituted carboxylates .
Scientific Research Applications
Rhodium(II) acetate has a wide range of scientific research applications:
Chemistry: It is used as a catalyst for various organic transformations, including cyclopropanation, oxidation, and insertion reactions
Medicine: It is explored for its potential in drug synthesis and development.
Industry: It is used in the functionalization of fullerenes into polymers and other industrial applications.
Mechanism of Action
Rhodium(II) acetate exerts its effects primarily through its catalytic properties. It forms rhodium carbenoids when treated with diazo compounds, enabling various organic transformations . The molecular targets and pathways involved include the activation of C-H and X-H bonds, facilitating the formation of new chemical bonds .
Comparison with Similar Compounds
Rhodium(II) acetate is often compared with other transition metal carboxylates, such as:
Copper(II) acetate: Similar structure but less reactive in aqueous solutions.
Chromium(II) acetate: Similar structure but different catalytic properties.
This compound is unique due to its high reactivity and versatility in catalyzing a wide range of organic reactions .
Properties
IUPAC Name |
rhodium(2+);diacetate | |
---|---|---|
Source | PubChem | |
URL | https://pubchem.ncbi.nlm.nih.gov | |
Description | Data deposited in or computed by PubChem | |
InChI |
InChI=1S/2C2H4O2.Rh/c2*1-2(3)4;/h2*1H3,(H,3,4);/q;;+2/p-2 | |
Source | PubChem | |
URL | https://pubchem.ncbi.nlm.nih.gov | |
Description | Data deposited in or computed by PubChem | |
InChI Key |
ITDJKCJYYAQMRO-UHFFFAOYSA-L | |
Source | PubChem | |
URL | https://pubchem.ncbi.nlm.nih.gov | |
Description | Data deposited in or computed by PubChem | |
Canonical SMILES |
CC(=O)[O-].CC(=O)[O-].[Rh+2] | |
Source | PubChem | |
URL | https://pubchem.ncbi.nlm.nih.gov | |
Description | Data deposited in or computed by PubChem | |
Molecular Formula |
C4H6O4Rh | |
Source | PubChem | |
URL | https://pubchem.ncbi.nlm.nih.gov | |
Description | Data deposited in or computed by PubChem | |
DSSTOX Substance ID |
DTXSID30203567 | |
Record name | Acetic acid, rhodium(2+) salt | |
Source | EPA DSSTox | |
URL | https://comptox.epa.gov/dashboard/DTXSID30203567 | |
Description | DSSTox provides a high quality public chemistry resource for supporting improved predictive toxicology. | |
Molecular Weight |
220.99 g/mol | |
Source | PubChem | |
URL | https://pubchem.ncbi.nlm.nih.gov | |
Description | Data deposited in or computed by PubChem | |
Physical Description |
Green powder; Insoluble in water; [Alfa Aesar MSDS] | |
Record name | Rhodium(II) acetate dimer | |
Source | Haz-Map, Information on Hazardous Chemicals and Occupational Diseases | |
URL | https://haz-map.com/Agents/17098 | |
Description | Haz-Map® is an occupational health database designed for health and safety professionals and for consumers seeking information about the adverse effects of workplace exposures to chemical and biological agents. | |
Explanation | Copyright (c) 2022 Haz-Map(R). All rights reserved. Unless otherwise indicated, all materials from Haz-Map are copyrighted by Haz-Map(R). No part of these materials, either text or image may be used for any purpose other than for personal use. Therefore, reproduction, modification, storage in a retrieval system or retransmission, in any form or by any means, electronic, mechanical or otherwise, for reasons other than personal use, is strictly prohibited without prior written permission. | |
CAS No. |
5503-41-3, 15956-28-2 | |
Record name | Acetic acid, rhodium(2+) salt | |
Source | EPA DSSTox | |
URL | https://comptox.epa.gov/dashboard/DTXSID30203567 | |
Description | DSSTox provides a high quality public chemistry resource for supporting improved predictive toxicology. | |
Record name | Rhodium (II) acetate | |
Source | European Chemicals Agency (ECHA) | |
URL | https://echa.europa.eu/substance-information/-/substanceinfo/100.024.403 | |
Description | The European Chemicals Agency (ECHA) is an agency of the European Union which is the driving force among regulatory authorities in implementing the EU's groundbreaking chemicals legislation for the benefit of human health and the environment as well as for innovation and competitiveness. | |
Explanation | Use of the information, documents and data from the ECHA website is subject to the terms and conditions of this Legal Notice, and subject to other binding limitations provided for under applicable law, the information, documents and data made available on the ECHA website may be reproduced, distributed and/or used, totally or in part, for non-commercial purposes provided that ECHA is acknowledged as the source: "Source: European Chemicals Agency, http://echa.europa.eu/". Such acknowledgement must be included in each copy of the material. ECHA permits and encourages organisations and individuals to create links to the ECHA website under the following cumulative conditions: Links can only be made to webpages that provide a link to the Legal Notice page. | |
Record name | Tetrakis[μ-(acetato-O:O')]dirhodium | |
Source | European Chemicals Agency (ECHA) | |
URL | https://echa.europa.eu/substance-information/-/substanceinfo/100.036.425 | |
Description | The European Chemicals Agency (ECHA) is an agency of the European Union which is the driving force among regulatory authorities in implementing the EU's groundbreaking chemicals legislation for the benefit of human health and the environment as well as for innovation and competitiveness. | |
Explanation | Use of the information, documents and data from the ECHA website is subject to the terms and conditions of this Legal Notice, and subject to other binding limitations provided for under applicable law, the information, documents and data made available on the ECHA website may be reproduced, distributed and/or used, totally or in part, for non-commercial purposes provided that ECHA is acknowledged as the source: "Source: European Chemicals Agency, http://echa.europa.eu/". Such acknowledgement must be included in each copy of the material. ECHA permits and encourages organisations and individuals to create links to the ECHA website under the following cumulative conditions: Links can only be made to webpages that provide a link to the Legal Notice page. | |
Retrosynthesis Analysis
AI-Powered Synthesis Planning: Our tool employs the Template_relevance Pistachio, Template_relevance Bkms_metabolic, Template_relevance Pistachio_ringbreaker, Template_relevance Reaxys, Template_relevance Reaxys_biocatalysis model, leveraging a vast database of chemical reactions to predict feasible synthetic routes.
One-Step Synthesis Focus: Specifically designed for one-step synthesis, it provides concise and direct routes for your target compounds, streamlining the synthesis process.
Accurate Predictions: Utilizing the extensive PISTACHIO, BKMS_METABOLIC, PISTACHIO_RINGBREAKER, REAXYS, REAXYS_BIOCATALYSIS database, our tool offers high-accuracy predictions, reflecting the latest in chemical research and data.
Strategy Settings
Precursor scoring | Relevance Heuristic |
---|---|
Min. plausibility | 0.01 |
Model | Template_relevance |
Template Set | Pistachio/Bkms_metabolic/Pistachio_ringbreaker/Reaxys/Reaxys_biocatalysis |
Top-N result to add to graph | 6 |
Feasible Synthetic Routes
Disclaimer and Information on In-Vitro Research Products
Please be aware that all articles and product information presented on BenchChem are intended solely for informational purposes. The products available for purchase on BenchChem are specifically designed for in-vitro studies, which are conducted outside of living organisms. In-vitro studies, derived from the Latin term "in glass," involve experiments performed in controlled laboratory settings using cells or tissues. It is important to note that these products are not categorized as medicines or drugs, and they have not received approval from the FDA for the prevention, treatment, or cure of any medical condition, ailment, or disease. We must emphasize that any form of bodily introduction of these products into humans or animals is strictly prohibited by law. It is essential to adhere to these guidelines to ensure compliance with legal and ethical standards in research and experimentation.