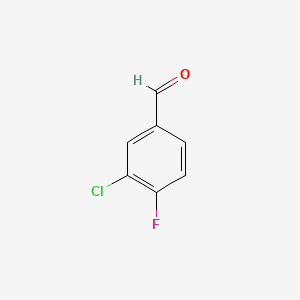
3-Chloro-4-fluorobenzaldehyde
Overview
Description
3-Chloro-4-fluorobenzaldehyde is an organic compound with the molecular formula C7H4ClFO. It is a derivative of benzaldehyde, where the hydrogen atoms at positions 3 and 4 on the benzene ring are replaced by chlorine and fluorine atoms, respectively. This compound is a valuable intermediate in organic synthesis, particularly in the pharmaceutical and agrochemical industries .
Preparation Methods
Synthetic Routes and Reaction Conditions: 3-Chloro-4-fluorobenzaldehyde can be synthesized through a halogen-exchange reaction. One common method involves the reaction of 4-chlorobenzaldehyde with an alkali fluoride in a dipolar aprotic solvent, such as dimethyl sulfoxide (DMSO) or dimethylformamide (DMF). The reaction typically proceeds at elevated temperatures to facilitate the exchange of the chlorine atom with a fluorine atom .
Industrial Production Methods: In industrial settings, the production of this compound follows similar synthetic routes but on a larger scale. The process involves the use of continuous flow reactors to ensure consistent product quality and yield. The reaction conditions are optimized to minimize by-products and maximize the efficiency of the halogen-exchange reaction .
Chemical Reactions Analysis
Types of Reactions: 3-Chloro-4-fluorobenzaldehyde undergoes various chemical reactions, including:
Nucleophilic Aromatic Substitution: The compound can participate in nucleophilic aromatic substitution reactions, where the chlorine or fluorine atom is replaced by a nucleophile.
Oxidation: The aldehyde group can be oxidized to form the corresponding carboxylic acid.
Reduction: The aldehyde group can be reduced to form the corresponding alcohol.
Common Reagents and Conditions:
Nucleophilic Aromatic Substitution: Strong nucleophiles such as sodium methoxide or potassium tert-butoxide are commonly used under basic conditions.
Oxidation: Oxidizing agents like potassium permanganate or chromium trioxide are used under acidic or basic conditions.
Reduction: Reducing agents such as sodium borohydride or lithium aluminum hydride are employed under mild conditions.
Major Products Formed:
Nucleophilic Aromatic Substitution: Products depend on the nucleophile used, such as methoxy or tert-butoxy derivatives.
Oxidation: 3-Chloro-4-fluorobenzoic acid.
Reduction: 3-Chloro-4-fluorobenzyl alcohol.
Scientific Research Applications
3-Chloro-4-fluorobenzaldehyde is utilized in various scientific research applications, including:
Chemistry: It serves as a building block for the synthesis of more complex organic molecules, including pharmaceuticals and agrochemicals.
Biology: The compound is used in the synthesis of bioactive molecules that can interact with biological targets.
Medicine: It is a precursor in the synthesis of potential drug candidates, particularly those targeting specific enzymes or receptors.
Industry: The compound is used in the production of specialty chemicals and materials, including polymers and dyes
Mechanism of Action
The mechanism of action of 3-chloro-4-fluorobenzaldehyde is primarily related to its reactivity as an aldehyde. The compound can form Schiff bases through condensation reactions with amines, which are important intermediates in the synthesis of various bioactive molecules. Additionally, the presence of chlorine and fluorine atoms on the benzene ring can influence the compound’s reactivity and interaction with other molecules, making it a versatile intermediate in organic synthesis .
Comparison with Similar Compounds
3-Chlorobenzaldehyde: Similar structure but lacks the fluorine atom.
4-Fluorobenzaldehyde: Similar structure but lacks the chlorine atom.
3,4-Dichlorobenzaldehyde: Contains two chlorine atoms instead of one chlorine and one fluorine atom.
Comparison: 3-Chloro-4-fluorobenzaldehyde is unique due to the presence of both chlorine and fluorine atoms on the benzene ring. This dual substitution can enhance the compound’s reactivity and selectivity in various chemical reactions compared to its mono-substituted counterparts. The combination of these halogens can also influence the compound’s physical properties, such as boiling and melting points, making it a valuable intermediate in the synthesis of diverse organic molecules .
Properties
IUPAC Name |
3-chloro-4-fluorobenzaldehyde | |
---|---|---|
Source | PubChem | |
URL | https://pubchem.ncbi.nlm.nih.gov | |
Description | Data deposited in or computed by PubChem | |
InChI |
InChI=1S/C7H4ClFO/c8-6-3-5(4-10)1-2-7(6)9/h1-4H | |
Source | PubChem | |
URL | https://pubchem.ncbi.nlm.nih.gov | |
Description | Data deposited in or computed by PubChem | |
InChI Key |
GVORVQPNNSASDM-UHFFFAOYSA-N | |
Source | PubChem | |
URL | https://pubchem.ncbi.nlm.nih.gov | |
Description | Data deposited in or computed by PubChem | |
Canonical SMILES |
C1=CC(=C(C=C1C=O)Cl)F | |
Source | PubChem | |
URL | https://pubchem.ncbi.nlm.nih.gov | |
Description | Data deposited in or computed by PubChem | |
Molecular Formula |
C7H4ClFO | |
Source | PubChem | |
URL | https://pubchem.ncbi.nlm.nih.gov | |
Description | Data deposited in or computed by PubChem | |
DSSTOX Substance ID |
DTXSID80344010 | |
Record name | 3-Chloro-4-fluorobenzaldehyde | |
Source | EPA DSSTox | |
URL | https://comptox.epa.gov/dashboard/DTXSID80344010 | |
Description | DSSTox provides a high quality public chemistry resource for supporting improved predictive toxicology. | |
Molecular Weight |
158.56 g/mol | |
Source | PubChem | |
URL | https://pubchem.ncbi.nlm.nih.gov | |
Description | Data deposited in or computed by PubChem | |
CAS No. |
34328-61-5 | |
Record name | 3-Chloro-4-fluorobenzaldehyde | |
Source | CAS Common Chemistry | |
URL | https://commonchemistry.cas.org/detail?cas_rn=34328-61-5 | |
Description | CAS Common Chemistry is an open community resource for accessing chemical information. Nearly 500,000 chemical substances from CAS REGISTRY cover areas of community interest, including common and frequently regulated chemicals, and those relevant to high school and undergraduate chemistry classes. This chemical information, curated by our expert scientists, is provided in alignment with our mission as a division of the American Chemical Society. | |
Explanation | The data from CAS Common Chemistry is provided under a CC-BY-NC 4.0 license, unless otherwise stated. | |
Record name | 3-Chloro-4-fluorobenzaldehyde | |
Source | EPA DSSTox | |
URL | https://comptox.epa.gov/dashboard/DTXSID80344010 | |
Description | DSSTox provides a high quality public chemistry resource for supporting improved predictive toxicology. | |
Retrosynthesis Analysis
AI-Powered Synthesis Planning: Our tool employs the Template_relevance Pistachio, Template_relevance Bkms_metabolic, Template_relevance Pistachio_ringbreaker, Template_relevance Reaxys, Template_relevance Reaxys_biocatalysis model, leveraging a vast database of chemical reactions to predict feasible synthetic routes.
One-Step Synthesis Focus: Specifically designed for one-step synthesis, it provides concise and direct routes for your target compounds, streamlining the synthesis process.
Accurate Predictions: Utilizing the extensive PISTACHIO, BKMS_METABOLIC, PISTACHIO_RINGBREAKER, REAXYS, REAXYS_BIOCATALYSIS database, our tool offers high-accuracy predictions, reflecting the latest in chemical research and data.
Strategy Settings
Precursor scoring | Relevance Heuristic |
---|---|
Min. plausibility | 0.01 |
Model | Template_relevance |
Template Set | Pistachio/Bkms_metabolic/Pistachio_ringbreaker/Reaxys/Reaxys_biocatalysis |
Top-N result to add to graph | 6 |
Feasible Synthetic Routes
Disclaimer and Information on In-Vitro Research Products
Please be aware that all articles and product information presented on BenchChem are intended solely for informational purposes. The products available for purchase on BenchChem are specifically designed for in-vitro studies, which are conducted outside of living organisms. In-vitro studies, derived from the Latin term "in glass," involve experiments performed in controlled laboratory settings using cells or tissues. It is important to note that these products are not categorized as medicines or drugs, and they have not received approval from the FDA for the prevention, treatment, or cure of any medical condition, ailment, or disease. We must emphasize that any form of bodily introduction of these products into humans or animals is strictly prohibited by law. It is essential to adhere to these guidelines to ensure compliance with legal and ethical standards in research and experimentation.