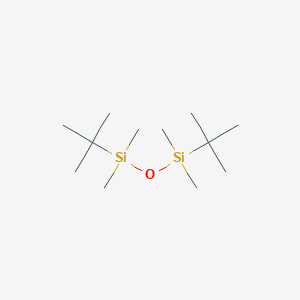
Disiloxane, 1,3-bis(1,1-dimethylethyl)-1,1,3,3-tetramethyl-
Overview
Description
Disiloxane, 1,3-bis(1,1-dimethylethyl)-1,1,3,3-tetramethyl- is a chemical compound belonging to the class of organosilicon compounds. It is characterized by the presence of two silicon atoms connected by an oxygen atom, with each silicon atom further bonded to two methyl groups and one tert-butyl group. This compound is known for its unique structural properties and is used in various industrial and research applications.
Preparation Methods
Synthetic Routes and Reaction Conditions: The synthesis of Disiloxane, 1,3-bis(1,1-dimethylethyl)-1,1,3,3-tetramethyl- typically involves the reaction of chlorosilanes with alcohols or silanols. One common method is the reaction of tert-butylchlorodimethylsilane with tert-butylsilanol under anhydrous conditions. The reaction is usually carried out in the presence of a base such as triethylamine to neutralize the hydrochloric acid formed during the reaction.
Industrial Production Methods: In industrial settings, the production of this compound may involve continuous flow processes where chlorosilanes and silanols are reacted in a controlled environment. The use of catalysts and optimized reaction conditions ensures high yield and purity of the final product.
Types of Reactions:
Oxidation: Disiloxane compounds can undergo oxidation reactions, typically forming silanols or siloxane oligomers.
Reduction: Reduction reactions are less common but can involve the conversion of disiloxane to silane derivatives.
Substitution: Substitution reactions can occur at the silicon atoms, where alkyl or aryl groups replace the existing substituents.
Common Reagents and Conditions:
Oxidation: Reagents such as hydrogen peroxide or peracids are commonly used.
Reduction: Metal hydrides like lithium aluminum hydride can be employed.
Substitution: Organometallic reagents such as Grignard reagents or organolithium compounds are often used.
Major Products Formed:
Oxidation: Silanols and siloxane oligomers.
Reduction: Silane derivatives.
Substitution: Various organosilicon compounds depending on the substituents introduced.
Scientific Research Applications
Disiloxane, 1,3-bis(1,1-dimethylethyl)-1,1,3,3-tetramethyl- finds applications in several scientific research fields:
Chemistry: Used as a precursor in the synthesis of more complex organosilicon compounds.
Biology: Employed in the development of biocompatible materials and drug delivery systems.
Medicine: Investigated for its potential use in medical devices and implants due to its biocompatibility.
Industry: Utilized in the production of silicone-based lubricants, sealants, and coatings.
Mechanism of Action
The mechanism of action of Disiloxane, 1,3-bis(1,1-dimethylethyl)-1,1,3,3-tetramethyl- involves its interaction with various molecular targets through its silicon-oxygen-silicon backbone. The compound can form stable bonds with other silicon or oxygen-containing molecules, leading to the formation of larger siloxane networks. This property is particularly useful in the development of silicone-based materials with desirable mechanical and chemical properties.
Comparison with Similar Compounds
Disiloxane: The simplest form of siloxane with only hydrogen atoms attached to the silicon atoms.
Hexamethyldisiloxane: A similar compound with six methyl groups attached to the silicon atoms.
Octamethylcyclotetrasiloxane: A cyclic siloxane with eight methyl groups and four silicon atoms.
Uniqueness: Disiloxane, 1,3-bis(1,1-dimethylethyl)-1,1,3,3-tetramethyl- is unique due to the presence of bulky tert-butyl groups, which provide steric hindrance and influence the compound’s reactivity and physical properties. This makes it particularly useful in applications where stability and resistance to degradation are important.
Properties
IUPAC Name |
tert-butyl-[tert-butyl(dimethyl)silyl]oxy-dimethylsilane | |
---|---|---|
Source | PubChem | |
URL | https://pubchem.ncbi.nlm.nih.gov | |
Description | Data deposited in or computed by PubChem | |
InChI |
InChI=1S/C12H30OSi2/c1-11(2,3)14(7,8)13-15(9,10)12(4,5)6/h1-10H3 | |
Source | PubChem | |
URL | https://pubchem.ncbi.nlm.nih.gov | |
Description | Data deposited in or computed by PubChem | |
InChI Key |
FGTJJHCZWOVVNH-UHFFFAOYSA-N | |
Source | PubChem | |
URL | https://pubchem.ncbi.nlm.nih.gov | |
Description | Data deposited in or computed by PubChem | |
Canonical SMILES |
CC(C)(C)[Si](C)(C)O[Si](C)(C)C(C)(C)C | |
Source | PubChem | |
URL | https://pubchem.ncbi.nlm.nih.gov | |
Description | Data deposited in or computed by PubChem | |
Molecular Formula |
C12H30OSi2 | |
Source | PubChem | |
URL | https://pubchem.ncbi.nlm.nih.gov | |
Description | Data deposited in or computed by PubChem | |
DSSTOX Substance ID |
DTXSID60343485 | |
Record name | TBDMS ether | |
Source | EPA DSSTox | |
URL | https://comptox.epa.gov/dashboard/DTXSID60343485 | |
Description | DSSTox provides a high quality public chemistry resource for supporting improved predictive toxicology. | |
Molecular Weight |
246.54 g/mol | |
Source | PubChem | |
URL | https://pubchem.ncbi.nlm.nih.gov | |
Description | Data deposited in or computed by PubChem | |
CAS No. |
67875-55-2 | |
Record name | TBDMS ether | |
Source | EPA DSSTox | |
URL | https://comptox.epa.gov/dashboard/DTXSID60343485 | |
Description | DSSTox provides a high quality public chemistry resource for supporting improved predictive toxicology. | |
Synthesis routes and methods I
Procedure details
Synthesis routes and methods II
Procedure details
Synthesis routes and methods III
Procedure details
Retrosynthesis Analysis
AI-Powered Synthesis Planning: Our tool employs the Template_relevance Pistachio, Template_relevance Bkms_metabolic, Template_relevance Pistachio_ringbreaker, Template_relevance Reaxys, Template_relevance Reaxys_biocatalysis model, leveraging a vast database of chemical reactions to predict feasible synthetic routes.
One-Step Synthesis Focus: Specifically designed for one-step synthesis, it provides concise and direct routes for your target compounds, streamlining the synthesis process.
Accurate Predictions: Utilizing the extensive PISTACHIO, BKMS_METABOLIC, PISTACHIO_RINGBREAKER, REAXYS, REAXYS_BIOCATALYSIS database, our tool offers high-accuracy predictions, reflecting the latest in chemical research and data.
Strategy Settings
Precursor scoring | Relevance Heuristic |
---|---|
Min. plausibility | 0.01 |
Model | Template_relevance |
Template Set | Pistachio/Bkms_metabolic/Pistachio_ringbreaker/Reaxys/Reaxys_biocatalysis |
Top-N result to add to graph | 6 |
Feasible Synthetic Routes
Disclaimer and Information on In-Vitro Research Products
Please be aware that all articles and product information presented on BenchChem are intended solely for informational purposes. The products available for purchase on BenchChem are specifically designed for in-vitro studies, which are conducted outside of living organisms. In-vitro studies, derived from the Latin term "in glass," involve experiments performed in controlled laboratory settings using cells or tissues. It is important to note that these products are not categorized as medicines or drugs, and they have not received approval from the FDA for the prevention, treatment, or cure of any medical condition, ailment, or disease. We must emphasize that any form of bodily introduction of these products into humans or animals is strictly prohibited by law. It is essential to adhere to these guidelines to ensure compliance with legal and ethical standards in research and experimentation.